Unit – 3
Fuels
Coal- Coal, is nothing but the primary fossil fuel, that is rich in carbon, coal is usually black or brown in colour, and occur in stratified sedimentary deposits.
The key difference between proximate and ultimate analysis of coal is that proximate analysis is the technique used to analyse the moisture content, ash content and fixed carbon of coal whereas ultimate analysis is the technique used to analyse the chemical composition of coal.
The technique of proximate analysis involves the determination of the different compounds present in a mixture. Ultimate analysis, on the other hand, involves the determination of the number and types of different chemical elements present in a particular compound. Therefore, these two techniques are related to each other.
Proximate analysis of coal is the process of determining the presence of different compounds and their amounts in coal. The technique of proximate analysis was developed by Henneberg and Stohmann (German scientists) in 1860. This analysis technique involves the partitioning of compounds into different categories depending on the chemical properties of these compounds. Mainly, there are six categories of compounds as moisture, ash, crude protein, crude lipid, crude fibre, and nitrogen-free extracts. In the process of proximate analysis of coal, the moisture content of coal, ash content of coal and the fixed carbon content of coal are determined.
The ultimate analysis of coal is the process of determining different chemical elements present in coal. This technique allows us to get more comprehensive results compared to the proximate analysis process.
In this analysis technique, we test moisture, ash, carbon, hydrogen, nitrogen, sulphur and oxygen content of the sample to determine the elemental composition of the coal sample. Therefore, each and every chemical element in the sample is analysed through chemical routes and then we can express the contents as percentages with respect to the total mass of the sample. Mostly, this analysis technique is useful in the coal and coke industry.
Calorific value of fuels
The calorific value is the value that shows the amount of heat liberated on complete combustion. For solid and liquid fuels, calorific value is expressed in kJ/kg, whereas for gaseous fuels it is expressed as kJ/m3 where m3 is normal cubic metre measured at NTP conditions i.e., at 0°C temperature and 760 mm Hg barometric pressure (1.01325 bar). Sometimes, calorific value of gaseous fuels can also be expressed as kJ per cubic metre expressed at STP conditions. STP (standard temperature and pressure) conditions are taken as 15°C and 760 mm Hg barometric pressure (1.01325 bar)
Bomb Calorimeter
Calorimeter, is a device that measures the heat developed when a chemical electrical or chemical reaction taking place, the device is also used to calculate the capacity of heat of materials.
The bomb calorimeter, consists of an enclosure that is surrounded by a liquid; however, the reaction occurs in the enclosure, the surrounding liquid may be water as it absorbs the heat produced in the reaction and thereby increases the temperature. If the weight and heat characteristics of the container is known, and the rise in temperature is measured, the total amount of heat can be calculated.
The design of a typical bomb calorimeter is shown below. The bomb is a steel reaction vessel, where the material to be analysed is deposited. The steel bomb vessel is placed inside a bucket that is filled with water, the temperature of the bucket is kept constant by using a stirrer and a heater. The variation in temperature is observed on a thermometer fitted with a magnifying glass, and the accurate reading are recorded. The presence of slots at the top of the steel bomb that allows the ignition wires and oxygen supply to enter the vessel as both these factors are critical for the reaction. Heat losses are minimized by inserting an air space between the bucket and an exterior insulating jacket. When an electric current pass through the ignition coil, a combustion reaction occurs. The heat released from the sample is largely absorbed by the water, which results in an increase in temperature. Bomb calorimeters have been developed to the point that heats of combustion of organic materials can be measured with results reproducible within 0.01 percent.
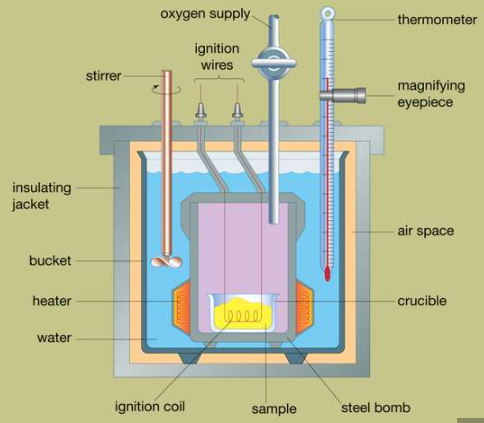
Fig: 1 Cross section of a typical bomb calorimeter
High Calorific value (HCV)
It is the heat liberated by the combustion of the fuel, the heat required to evaporate the water should be neglected, is called the High Calorific value. It is the maximum heat liberated by complete combustion of fuel; HCV is also called gross calorific value.
If we subtract from the higher calorific value, an amount of heat required to evaporate the water formed, we get Lower Calorific Value (LCV) or Net Calorific Value (LCV) or Net Calorific Value of the fuel.
No definite agreement is to be found in the literature on fuel as to whether the lower calorific value shall be found simply by subtracting latent heat of steam or both the latent heat and sensible heat in cooling from 100°C, from the gross calorific value of the fuel; in the latter case fixing the temperature becomes mandatory to which the products are finally reduced. In literature the net calorific value of the fuel is obtained by subtracting from higher calorific value the amount 2466 kJ/kg (latent heat of dry and saturated steam at STP (15°C) conditions) (amount of water formed because of the combustion of 1 kg fuel.)
Refining of petroleum
The crude petroleum oil is a complex mixture of solid liquid and gaseous hydrocarbons
Before petroleum can be used for various purposes, it is refined or purified, the process of separating crude oil into more useful fractions is called refining. The refining of petroleum into different fractions is based on the fact that different fractions have different boiling points. The refining of petroleum is carried out in an oil refinery.
The crude petroleum oil extracted from the oil well is transferred to an oil refinery through pipes. In the oil refinery crude petroleum oil is refined into useful fractions. The separation of petroleum into different fractions is done by fractional distillation. The various useful fraction that are obtained from refining of petroleum are Petroleum gas, fractions.
Cracking, is a process in petroleum refining. In this process heavy molecules of hydrocarbon are broken down into lighter molecules by heat, pressure and sometimes catalysts. It is a very important process for the commercial production of gasoline and diesel oil.
The process of cracking yields light oil, middle oils that is used in diesel fuel, heavy residual oils and also solid carbonaceous product like coke etc. Gases such as methane, ethane. Ethylene, propane, propylene and butylene are also produced during cracking. The end product formed in the process can directly go into fuel blending or can be subjected to future process, until oils of desired weight are produced. The gases can be used in the refinery’s fuel system, Certain raw materials are also produced from the petrochemical plants, where a large number of end products can be obtained from them like synthetic rubber, plastic and also agricultural products.
Petrol
It is a volatile flammable liquid, that consists of a mixture of hydrocarbons namely heptane, hexane and octane. They are mainly obtained from petroleum and used as a solvent and fuel for internal combustion engines. Petrol also contains additives such as antiknock compounds and corrosion inhibitors.
Diesel
Is a fuel where the chemical energy stored in the fuel is converted into mechanical energy, any internal combustion engine, where air is compressed to very high temperatures to ignite the diesel fuel injected into the cylinder. The piston is actuated by combustion and expansion, the mechanical energy can to used to drive freight expansion, The tractors and marine vessels. A limited number of automobiles also are diesel-powered, as are some electric-power generator sets.
Octane and Cetane numbers
Octane Number and Cetane Number are the standards to measure the tendency of fuel to ignite spontaneously. The performance of gasoline is measured by the Octane number on the other hand the cetane number measures the performance of diesel. The reason why petrol can’t be used in diesel engine and diesel can’t be used in a petrol engine is that when the fuel that has high octane number will have low cetane number and high cetane number fuel has low octane number.
As per the Standard operating conditions the Octane number of a fuel defines the percentage of Iso-butene present in a mixture of Iso-octane and heptane. When used in a gasoline engine the Octane rating signifies the ability to resist auto ignition. As air and fuel are compressed together, gasoline tends to ignite a spark at the end of compression by a spark plug. If gasoline with low octane number is used it creates problems during ignition and tends to adapt to auto combustion easily due to excess of heat and pressure effects on the other hand, fuels that have high octane value takes more time to burn but provides maximum efficiency to the gasoline engine.
Cetane is a type of chemical compound known as a Hexadecane. Cetane number is opposite to octane number, and measures how quickly the engine burns inside a compressed engine. Cetane compounds tend to ignite spontaneously under compression, therefore they are assigned as cetane number of hundred. Cetane number of a fuel can be defined as the percentage volume of n-hexadecane in the mixture of n-hexadecane and 1-methylnaphthalene which is responsible to provide ignition delay period.
Knocking and Anti-knocking
Knocking, this usually occurs in an internal combustion engine, where sharp sounds occurs due to the premature combustion from the part of the compressed air-fuel mixture present in the cylinder. When an engine functions smoothly in the combustion chamber, the charge burns with the flame front smoothly progressing from the point of ignition. In some cases, depending upon the composition of the fuel, at high compression ratios, some of the charge may ignite spontaneously ahead of the flame front resulting in burning in an uncontrolled manner that produces pressure waves of high frequency. The waves are responsible for forcing parts of the engine to vibrate and produce audible sound.
The effects of Knocking can cause overheating of the spark-plug points, erosion of the combustion chamber surface, and rough, inefficient operation.
An antiknock agent is a gasoline additive that is used to reduce knocking that occurs in engines, the fuels octane number is increased by increasing the temperature and pressure at which auto-ignition occurs. As the gasoline engine developed, gasoline and the engine were harmonized to attain the best possible matching of characteristics. One of the important characteristics of gasoline is it is volatile in nature and antiknock quality, Volatility is a measure of the ease of vaporization of gasoline, Gasoline also helps to start engines in cold weather and to avoid vapour lock in hot weather conditions.
Fischer-Tropsch reaction,
The process was first used in Germany in 1940 for producing liquid and gaseous hydrocarbon fuels, such as gasoil, the method has got its name from German chemists Franz Fischer and Hans tropsch. The reaction consists of a collection of chemical reactions where conversion of a mixture of carbon monoxide and hydrogen is converted into liquid hydrocarbons, the reaction occurs in the presence of metal catalysts and at a temperature of 150–300 °C (302–572 °F) and pressures of one to several tens of atmospheres.
- Reactions involving substitution, addition, elimination, oxidation-reduction
These are the four "prototypical" organic chemistry reactions, though several others which can be categorized as one of these are generally referred to by other names. A glance at these reactions and these question for each would arise: what bonds are formed, and what bonds are broken.
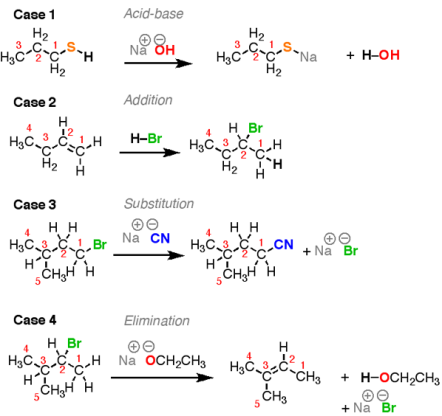
Fig. 2: Four general reactions in Organic Chemistry.
Which of the bonds are broken, and which bonds are formed? A rearrangement reaction is not demonstrated.
At least 80% of the reactions in organic chemistry fall into one of these four categories. The sooner the bond formation and breakage are recognized, a clearer picture is visible. A fifth reaction is also discussed later in the chapter: rearrangement reactions.
Acid/Base Reactions
Another important category of organic reactions is straight-forward Bronsted–Lowry acid-base reactions.
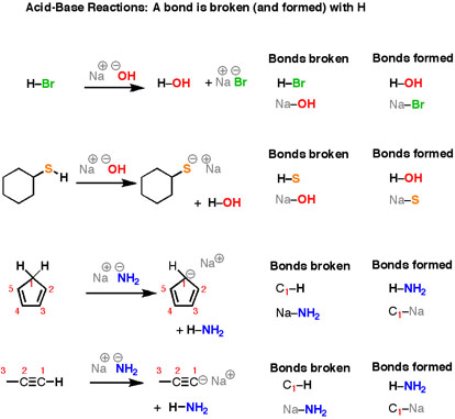
Fig.3: Acid/Base reactions
It can be noticed that the reactions look different on the surface – all those different structures! – but the basic plot of each reaction is the same. We are breaking an H-(atom) bond and forming an H-(atom) bond. At the same time, two “leftover” partners connect together to form a salt, composed of two oppositely-charged ions. Let’s look at that last reaction in more detail. Here, the breaking of a C-H bond and an (ionic) Na-NH2 bond, and forming an N-H bond as well as an (ionic) C-Na bond.
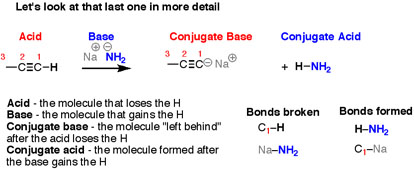
Fig. 4: There are four “actors” in this reaction – as there are in every acid-base reaction –
- In the reaction, the bond to H is breaking is the acid.
- The next level where, where the bond to H is forming is the base
- The product that is formed, is when the bond to H is broken it is called the conjugate base.
- The product that is formed, when the bond to H is formed is called the conjugate acid.
When, the reverse of the previous reaction is looked into carefully, the breaking of a bond to H and forming a bond to H happens, but after swapping everything, the breaking of N-H and C-Na takes place, and forming of N-Na and C-H occurs.
It’s still an acid-base reaction.
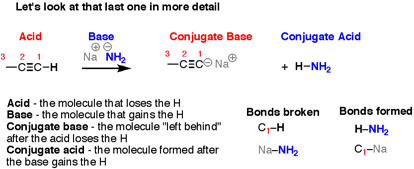
Fig.5: The four reactions that take place in this reaction, Acid-Base-Conjugate Base-Conjugate Acid.
However, experiment tells us that this reaction does not happen to any appreciable extent.
In this acid/base reaction, which are the bonds that are broken and which bonds are formed, if any?
(CH3−CH2)3N+HCl→(CH3−CH2)3NH++Cl−onumber (27.1.1) (27.1.1) (CH3−CH2)3N+HCl→(CH3−CH2)3NH++Cl−onumber
- Addition Reactions
When an alkene (or alkyne) is taken and certain types of reagents are added to them, the resulting reaction is shown. If any bonds can be recognized, the bonds formed and broken should be recognised.
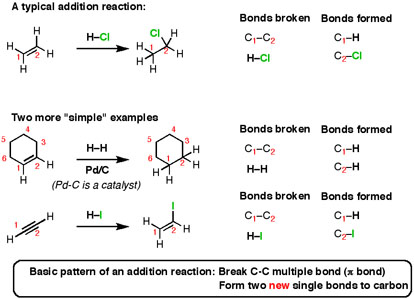
Fig.6: The reaction shows an Addition reaction, to an Alkene a suitable reagent is added, the C-C multiple bonds are broken and two new single carbon bonds are formed.
Here’s the basic pattern: break a C-C multiple bond (also called a π bond) and form two new single bonds (“σ-bonds” to carbon). This reaction is called an “addition reaction” and it’s a typical type of common reaction. Note that the reaction occurs only at the carbons that are a part of a multiple bond – nothing else on the molecule gets affected.
In this addition reaction, the question arises as to, which bonds are broken and which are formed, if any?
CH3−C(=O) −CH3−→−−−−H+/H2OLiAlH4CH3−CH(OH)−CH3onumber (27.1.2)(27.1.2)CH3−C(=O)−CH3→H+/H2OLiAlH4CH3−CH(OH)−CH3onumber
Note: This reaction could be described as a (nucleophilic) addition reaction or a reduction.
Substitution Reaction
Here are three examples of nucleophilic substitution reactions.
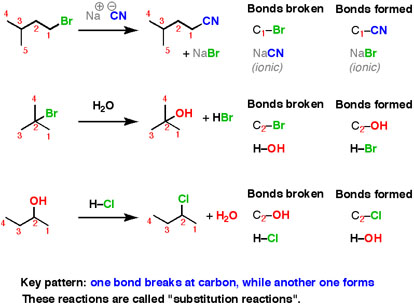
Fig.7: An example of nucleophilic substitution reactions.
In each case, we are breaking a bond at carbon, and forming a new bond at carbon. This is a very common pattern for reactions in organic chemistry, some interesting results are obtained from that experiments, most of the information we don’t get by thinking about what happens next and predict it.
In this substitution reaction, which bonds are broken and with are formed, if any?
CH3−C(=O)−OH+CH3−OH−→−−−−−− Δ and reflux H2SO4CH3−C(=O)−O−CH3+H2Oonumber(27.1.3)(27.1.3)CH3−C(=O)−OH+CH3−OH→ Δ and reflux H2SO4CH3−C(=O)−O−CH3+H2Oonumber
Note: This reaction would usually be called a condensation reaction.
Elimination Reactions
Let’s look at two simple cases. One important thing to keep in mind is that, although you see “Na OCH3” written here, the “Na+” (sodium) is not important for our purposes. It could alternatively be K+ (potassium) or Li+ (lithium). It’s just balancing the negative charge on the oxygen. When you take an alkyl halide and add a strong base (such as NaOCH3NaOCH3 or NaOCH2CH3NaOCH2CH3) a reaction occurs. See if you can recognize the bonds broken and formed.
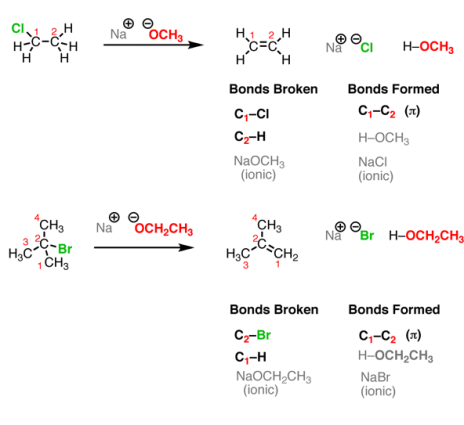
Fig.8: Elimination Reactions, these reactions are the reverse of addition reactions.
This reaction results in the formation of a new C-C double bond (π bond) and breaking of two single bonds to carbon (in such cases, one of them is H and the other is a halide such as Cl or Br). However, a new bond is formed between H and the O. So, in this case, this reaction incorporates a pattern we have already seen before – an acid-base reaction. Finally, a salt is formed in this reaction. But since the prime interest here is the organic product (that is, the one containing carbon), however, the salt by-product is not written in some reaction schemes, but that doesn’t mean that it’s not presents there.
NOTE
The three events in the elimination reaction of Fig (formation of C-C π bond, and breaking of two adjacent carbon sigma bonds) are the exactly opposite to the addition reactions discussed before.
Another example of an elimination reaction, is when 2-bromopropane is heated under reflux with a concentrated solution of sodium or potassium hydroxide in ethanol. Heating under reflux involves the heating with a condenser placed vertically in the flask to avoid loss of volatile liquids. The product formed from this reaction is Propene is and, because this is a gas, it passes through the condenser and is be collected.
Everything else that is present (including anything formed in the alternative substitution reaction) will be trapped in the flask.
In this elimination reaction, which bonds are broken and with are formed, if any?
CH3−CH(OH)−CH3−→−−−−K2Cr2O7H2SO4CH3−C(=O)−CH3onumber(27.1.4)(27.1.4)CH3−CH(OH)−CH3→K2Cr2O7H2SO4CH3−C(=O)−CH3onumber
Note: This reaction can also be described an oxidation reaction.
- Rearrangement Reactions
Rearrangement reactions can accompany many of the reactions that were previously covered such as substitution, addition, and elimination reactions. These reactions are comparatively very rare. In fact, however when closely looked, sometimes it’s a fact that few rearrangement reactions have occurred and have been missed or gone unnoticed. Let’s look at a substitution reaction first.
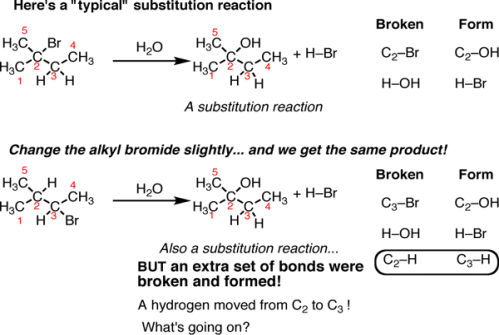
Fig.9: A typical substitution reaction, the C-Br bond is broken and a C-OH bond is formed.
On the top is a “typical” substitution reaction: we are taking an alkyl halide and adding water. The C-Br bond is broken and a C-OH bond is formed. If we look at the table on the right a typical pattern of substitution reactions is followed. However, if we change one thing about this alkyl halide – move the bromine to C-3 instead of C-2 – subsequently in this reaction a different product emerges. This is also a substitution reaction (where Br is replaced with OH) but it’s on a different carbon. when noticed closely, there are actually 3 bonds broken and 3 bonds formed. The C2-H bond broke and the C3-H bond formed.
In this Rearrangement reaction, which bonds are broken and with are formed, if any?
CH3−CH2−CH2−C(OH)=CH2→CH3−CH2−CH2−C(=O)−CH3onumber(27.1.5)(27.1.5)CH3−CH2−CH2−C(OH)=CH2→CH3−CH2−CH2−C(=O)−CH3onumber
Note: This reaction could also be described an oxidation reaction.
- Oxidation and reduction reactions
We find examples of oxidation-reduction or redox reactions almost every time we analyse the reactions used as sources of either heat or work. When natural gas burns, for example, an oxidation-reduction reaction occurs that releases more than 800 kJ/mol of energy.
CH4(g) + 2 O2(g) CO2(g) + 2 H2O(g)
The best example that happens within our bodies, is a sequence of oxidation-reduction reactions that occur to burn sugars, such as glucose (C6H12O6) and the fatty acids in the fats that we eat.
C6H12O6(aq) + 6 O2(g) ![]() |
CH3(CH2)16CO2H(aq) + 26 O2(g) ![]() |
These reactions can also be used as a source of energy, however, to find examples of oxidation-reduction reactions. Silver metal, for example, is oxidized when it comes in contact with trace quantities of H2S or SO2 in the atmosphere or foods, such as eggs, that are rich in sulfur compounds.
4 Ag(s) + 2 H2S(g) + O2(g) 2 Ag2S(s) + 2 H2O(g)
Surprisingly, the film of Ag2S that is formed collects on the metal surface and forms a protective coating that slows down further oxidation of the silver metal.
The given example of tarnishing of silver is just one of the reactions that occur in a broad class of oxidation-reduction reactions that come under the general heading of corrosion. Another example that occurs in a series of reactions when iron or steel rusts. When iron is heated, it reacts with oxygen to form a mixture of iron (II) and iron (III) oxides.
2 Fe(s) + O2(g) ![]() |
2 Fe(s) + 3 O2(g) 2 Fe2O3(s) |
Molten iron even reacts with water to form an aqueous solution of Fe2+ ions and H2 gas.
Fe(l) + 2 H2O(l) Fe2+(aq) + 2 OH-(aq) + H2(g)
At room temperature, however, all three of these reactions are so slow they can be easily ignored.
Iron can only corrode at room temperature in the presence of both water and oxygen. In the sequence of this reaction, the iron gets oxidized to give a hydrated form of iron (II) oxide.
2 Fe(s) + O2(aq) + 2 H2O(l) 2 FeO H2O(s)
Because iron (II) oxide has the same empirical formula as Fe (OH)2, usually iron (II), is mistaken and also called as ferrous hydroxide. The FeO H2O formed in this reaction is further oxidized by O2 dissolved in water to give a hydrated form of iron (III), or ferric oxide.
4 FeO H2O(s) + O2(aq) + 2 H2O(l) 2 Fe2O3 3 H2O(s)
In a further complicated reaction, FeO H2O formed at the metal surface combines with Fe2O3 3 H2O to give a hydrated form of magnetic iron oxide (Fe3O4) shown in the reaction below.
FeO H2O(s) + Fe2O3 3 H2O(s) Fe3O4 n H2O(s)
As these reactions only can occur in the presence of both water and oxygen, cars tend to rust easily wherever water collects. Furthermore, because the simplest way of preventing iron from rusting is to coat the metal surface so that it doesn't come in contact with water, cars were originally painted for only one reason to slow down the formation of rust.
Diels-Alder Reaction (Nobel Prize in 1950)
- The Diels-Alder reaction is a conjugate addition reaction of a conjugated diene to an alkene (the dienophile) to produce a cyclohexene.
- The simplest example is the reaction of 1,3-butadiene with ethene to form cyclohexene:

- The analogous reaction of 1,3-butadiene with ethyne to form 1,4-cyclohexadiene is also known:
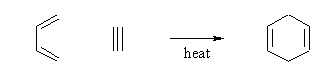
- Since the reaction forms a cyclic product, via a cyclic transition state, it can also be described as a "cycloaddition".
- The reaction is a concerted process:
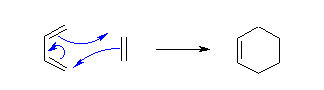
Due to the high degree of regio- and stereoselectivity (due to the concerted mechanism), the Diels-Alder reaction is a very powerful reaction and is popularly used in synthetic organic chemistry.
- The reaction is usually thermodynamically favourable due to the conversion of 2 π-bonds into 2 new stronger σ-bonds.
- The two reactions shown above require harsh reaction conditions, but the normal Diels-Alder reaction is favoured by electron withdrawing groups on the electrophilic dienophile and by electron donating groups on the nucleophilic diene.
- Some common examples of the components are shown below:
Dienes | ![]() |
Dienophiles | ![]() |
Stereoselectivity:
- The Diels-Alder reaction is stereospecific with respect to both the diene and the dienophile.
- Addition is sync on both components (bonds form from same species at the same time)
- This is illustrated by the examples below where you should compare the relative positions substituents in the JSMOL images:
Fig.10: The picture shows the relative positions of dienophile and diene substituents.
Cyclic dienes can give stereoisomeric products depending on whether the dienophile lies under or away from the diene in the transition state. The endo product is usually the major product (due to kinetic control)
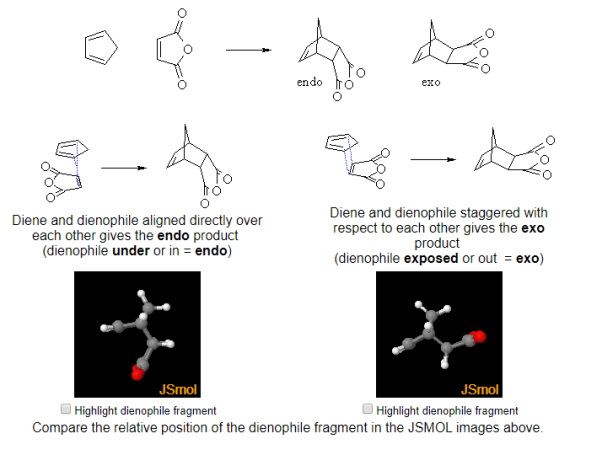
Fig.11: Cyclic dienes can give stereoisomeric products depending on whether the dienophile lies under or away from the diene in the transition state.
- Epoxide ring openings reactions
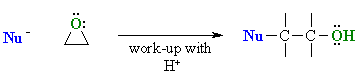
Reaction type: Nucleophilic Substitution
Summary
- Epoxides are much more reactive than simple ethers due to ring strain.
- The Nucleophiles attack the electrophilic C of the C-O bond causing it to break, resulting in ring opening.
- The Opening the of the ring eases the ring strain.
- The products formed are typically 2-substituted alcohols.
- Epoxides are known to react with a large range of nucleophiles.
Depending on how a nucleophile reacts, there are two possible general scenarios:
- The Stronger nucleophiles (anionic e.g. Alkoxides, Grignard reagents etc.)

- Examples of such nucleophilic systems are: RMgX, RLi, RC≡CM, LiAlH4, NaBH4
- The Weaker nucleophiles (neutral e.g. water) can react with epoxides in the presence of acid catalysts.

- Examples of such nucleophilic systems are: H2O, ROH, R-NH2
Structural Isomers and Stereo Isomers
Isomerism
Two or more compounds having the same molecular formula but different physical and chemical properties are called isomers and the phenomenon is known as isomerism. There are two types of isomerism
- Structural isomerism.
- Stereoisomerism.
Structural isomerism
Those compounds that have the same molecular formula but have different structures are called as structural isomers. Structural isomerism is further divided into six subcategories.
- Chain isomerism
The compounds that have same molecular formula but differ in carbon skeletons are called as chain isomers and the phenomenon is known as Chain isomerism.
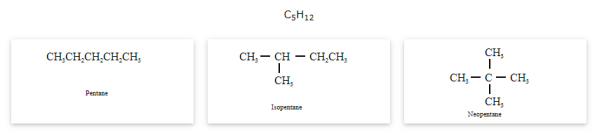
Position isomerism
The compounds that have same molecular formula but are different with respect to the position of the multiple bond (double or triple bond) or functional group are called as position isomers and the phenomenon is known as position isomerism.
Functional isomerism
The compounds that have same molecular formula but differ in their functional groups are called functional isomers and the phenomenon is called functional isomerism.

General formula for ether is R-O-R' where R and R' are different (or similar) carbon chains.
Metamerism
The compounds that have same formula but differ in alkyl chains on either side of the functional group are called as metamers and the phenomenon is known as metamerism.

Notice that the above compounds are also called as position isomers.
Tautomerism
Tautomerism occurs due to the 1,3-migration of a hydrogen atom within the same molecule. The best example of tautomerism is keto-enol tautomerism, where one form contains the keto group (>C=O) and the other form contains the enol (en + ol) group.
Acetaldehyde and ethanol

You may note that in keto-enol tautomerism, enol form is usually negligible.
Ring-chain isomerism
The compounds that have the same molecular formula but one possesses an open chain while the others possess cyclic structures are called ring-chain isomers and the phenomenon is known as ring-chain isomerism.
Stereoisomerism
The compounds that have the same formula but are different in spatial arrangement of one atoms or group of atoms in space are known as stereoisomers and the phenomenon is called stereoisomerism. Stereoisomerism has the following two sub categories:
- Conformational isomerism
- Configurational isomerism
- Conformational Isomerism
Conformational isomers are those isomers where the relative positions of few atoms differ in the molecule in the three-dimensional space because of the rotation about sigma bonds.
- Configurational isomerism
Configurational isomerism is caused due to remaking and breaking of covalent bonds. They are further divided into:
- Geometrical isomerism
- Optical isomerism
Configuration, symmetry and chirality
In the context of stereochemistry, the term is restricted to the arrangements of atoms of a molecular entity in space that distinguishes stereoisomers, the isomerism between which is not due to confirmation differences.
- Absolute configuration
It is the spatial arrangement of the atoms of a chiral
Molecular entity (or group) and its stereo
Chemical description e.g. R or S.
- Relative configuration
The configuration of any stereo genic (asymmetric) centre with respect to any other stereo genic centre contained within the same molecular entity. Unlike
Absolute configuration, relative configuration is a reflection-invariant. Relative configuration, distinguishing diastereoisomers, may be denoted by the configurational descriptors R*R*, R*R* (or l) and R*R*, S*S* (or u) which means, respectively, that the two centres have similar or opposite configurations. For molecules that have more than two
Asymmetric centres the prefix rel- may be used in front of the name of one
Enantiomer where R and S have been used. If any centres have known
Absolute configuration then only R*R* and S*S* can be used for the relative configuration.
Symmetry and chirality
The symmetry of a molecule describes how its different parts relate to one another geometrically. Symmetry plays an important role in many areas of chemistry, with effects on:
- Physical properties: e.g. Dipole moment, chirality
- Spectroscopic properties: geometric equivalence of groups or nuclei, transition intensities
- Bonding interactions: the bonds need to overlap of atomic orbitals of correct symmetry
- Symmetry Operation: a spatial manipulation performed on a molecule that leaves it in a configuration identical to and superimposable upon the original configuration.
Symmetry Element: It is an axis, point or plane about which a symmetry operation is performed.
Point Group: It is a symbol that identifies all the symmetry elements present in a molecule.
Symmetry Elements and Operations |
Element | Operation | Symbol | A Notes |
None | Identity | E |
|
Proper | Rotate by | Cn |
|
Mirror plane | Reflection | Σ |
|
Inversion | Inversion | I |
|
Improper | Rotate by 360º/n, then reflect ^ to axis | Sn |
|
Effects on Molecular Properties |
- Chirality:
For a molecule to be chiral, both the functions Inversion (i) and Reflection(σ) must be absent. * Those Molecules that belong to groups Cn (including E) and Dn are chiral. * This is a small simplification. The most accurate requirement for chirality is the lack of any Sn element, as the appearance of these is difficult to figure out, however there are a series of increasingly precise shortcuts used to Spotting a Chiral Compound.
6.4 Enantiomers and Diastereomers
There are two types of STEREOISOMERS, enantiomers and diastereomers.
Enantiomers contain chiral centers that are non-superimposable & mirror images. They only come in pairs!
Diastereomers contain chiral centres which are non-superimposable but are NOT mirror images. There can be more than 2 depending on the number of stereocenters.
An easy way to remember enantiomers from diastereomers is to analyse and study the picture given below. In cases where there are 2 chiral centers, 4 stereoisomers are possible formed. Only the exact opposites (diagonal arrows) are enantiomers and they therefore have a mirror image that is not superimposable. The molecules with only one stereocenter that differs (parallel arrows) are diastereomers.
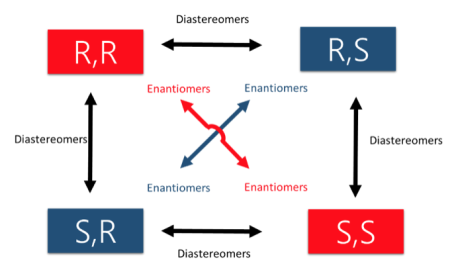
Fig.12: The picture shows two chiral centres, resulting in the formation of 4 stereoisomers. The diagonal arrows are enantiomers, their images are not superimposable though they are mirror images formed, the parallel arrows are diastereomers with only one stereocentre.
A biological example of this property can be viewed in, saccharide (or sugar) and below are the enantiomers and diastereomers of threose molecule.
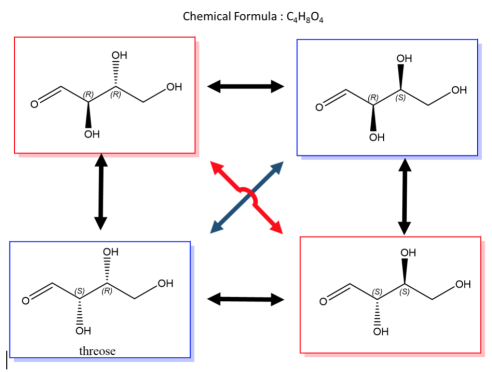
Fig.13: The picture shows a molecule of 5-DHT,an active form of Testosterone and has 7 stereocentres, Using the formula, 128 possible combinations are formed, only one is an enantiomer the rest are stereoisomers.
While enantiomers are found to only come in pairs, many diastereomers can exist without for a given molecule. Let’s take, 5-DHT for example, this compound is metabolically active form of testosterone and has 7 stereocenters, using the 2N rule for determining the number of stereoisomers, we get 128 possible combinations from the given formula. But only one of them is the enantiomer of 5DHT! The rest are diastereomers.
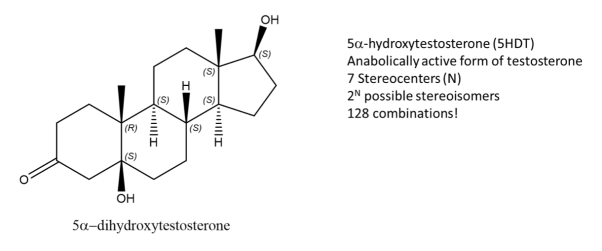
Fig.14: Structure of Testosterone, using the 2n rule, 128 combinations are formed.
Optical activity
The optical activity of a substance, is the tendency of a substance to rotate along the plane of polarization of a beam of light that is passed through it. (In a plane-polarized light, the vibrations of the electric field are confined to a single plane.) The intensity of optical activity of a substance is expressed in terms of a quantity, called specific rotation, the specific rotation is defined by an equation that is related to the angle through which the plane is rotated, the length of the light path through the sample, and the density of the sample (or its concentration if it is present in a solution). As the specific rotation depends upon the temperature and upon the wavelength of the light, these quantities also must be specified. The rotation is assigned a positive value if it is clockwise with respect to an observer facing the light source, negative if counter clockwise. A substance with a positive specific rotation is described as dextrorotatory and denoted by the prefix d or (+); a substance with a negative specific rotation is laevorotatory, designated by the prefix l or (-).
Optical activity was first discovered in quartz, crystals in by a French physicist in 1811, Francois Arago. Another French physicist, Jean-Baptiste Biot, found in 1815 that liquid solutions of tartaric acid or of sugar are optically active, as are liquid or vaporous turpentine. However, Louis Pasteur was the first to recognize that optical activity appears from the dissymmetric arrangement of atoms in the crystalline structures or also in individual molecules of certain compounds.
References:
- An Introduction to Coal Technology by Nobert Berkowitz
- Advanced Organic chemistry Part B: Reaction & Synthesis Francis.A.Cary Richard J. Sundberg
- Reaction Mechanisms in Organic chemistry – Dr. Mukul Ray