MODULE 5
LASERS
In 1917, some 9 years before linked quantum theory, Einstein postulated for thermodynamic details that the probability of spontaneous emission, A, was related to the probability of stimulated emission, B, of the relation
A / B = 8πhν3 / c3
From the development of the theory behind black body radiation, the energy density of equilibrium radiation per unit volume per unit frequency was known to be equal to
ρ (ν) = 8πhν3 / c3
Einstein argued that equilibrium would be possible and that the laws of thermodynamics obeyed, only if the relationship between the coefficients A and B had the value shown above.
This relationship was calculated by quantum mechanics in the mid-1920s. In recognition of Einstein's insight, the coefficients continued to be called Einstein's A and B coefficients.
Although applicable in many situations, coefficients A and B received particular attention in the period when lasers were developed. The nature of the coefficients is such that it is not possible to use radiation in a cavity to preferably raise the electrons to a higher state, producing the population inversion necessary for the action of the laser.
The particular relationship between the coefficients suggests that the presence of light to "pump" the electrons in the higher states will have the same probability of stimulating an already high electron to make the transition downward, so that the action of the laser cannot be achieved no two-tier system tier.
The achievement of the laser action was obtained by three-level systems, such as that of the helium-neon laser in which the population of the highest neon level could be reached by means of a non-radiative transfer of the pumpingenergy of the helium gas to neon atoms.
The implication of Einstein's coefficients A and B is that these two processes will occur at the same speed, so that population inversion cannot be achieved in a two-tier system like the one represented here.
In the helium-neon laser, for example, this limitation is overcome by collision transfer of helium gas to neon gas, obtaining the population inversion necessary for the action of the laser.
Laser - Population investment Excerpt from Spectroscopy Field Guide Under normal circumstances, the higher the energy level, the less populated it is with thermal energy.
In some circumstances (for example, the presence of a higher energy level that has a relatively long lifespan), a system can be built so that there are more atoms / molecules at a higher energy level than allowed under conditions of normal thermodynamic equilibrium
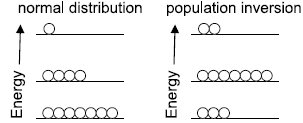
This arrangement is called population inversion. When there is a population inversion, a higher state system will eventually emit a photon of the correct wavelength and fall to the ground state.
However, this photon can stimulate the production of other photons of exactly the same wavelength due to the stimulated emission of radiation.
Therefore, many photons of the same wavelength (and phase, and other similar characteristics) can be generated in a short time.
This is the amplification of light by stimulated emission of radiation or LASER, generally seen in lowercase as a laser.
Lasers usually have a very narrow wavelength emission range. Many types of energy levels (electronic, vibratory, semiconductor bands) are used to produce lasers.
Many resources are active laser bases, including Cr3 + ions in ruby and alexandrite crystals, Ti3 + in sapphire, Nd3 + in garnet, and a variety of doped semiconductors.
Gases or gas mixtures such as He / Ne, Ar, Kr, CO2, N2, even Cu (g) can be dissolved. Some organic dyes can also be made to loosen; An advantage is that its laser action has a wider range of wavelengths (20-30 nm) and can be adjusted to a particular wavelength.
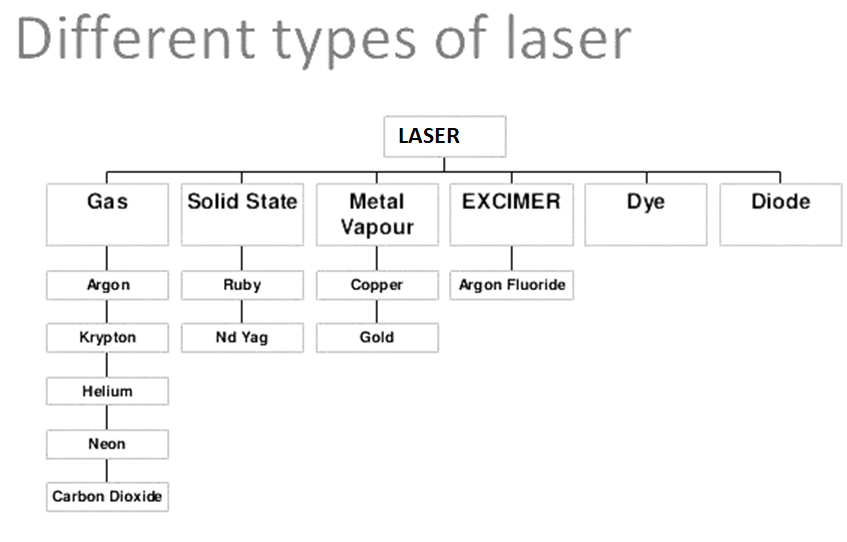
5.2.1 Helium Neon (He-Ne) Lasers:
The He-Ne laser was the first invented continuous wave (cw) laser. A few months after Maiman announced his invention of the pulsed ruby laser, Ali Javan and partners W. R. Bennet and D. R. Herriott announced their creation of a He-Ne cw laser.
This gas laser is a four-level laser that uses helium atoms to excite neon atoms. It is the atomic transitions in the neon that produce laser light.
The maximumusually used neon conversion in these lasers products red light at 632.8 nm. But these lasers can also produce both visible and UV and IR green and yellow light (Javan's first He-Ne worked on IR at 1152.3 nm).
Using highly reflective mirrors designed for one of these many
possible laser transitions, a given He-Ne output is operated at a single wavelength.
He-Ne lasers generally produce a few to tens of mW (milliwatts or 10-3 W) of power. They are not high power laser light sources.
Probably one of the most important characteristics of these lasers is that they are highly stable, both in terms of wavelength (mode stability) and intensity of light output (low fluctuation in power level).
For these reasons, He-Ne lasers are often used to stabilize other lasers. They are also used in applications such as holography, where mode stability is important.
Until the mid-1990s, He-Ne lasers were the dominant type of lasers produced for low-power applications, from range search to scanning, optical transmission, laser pointers, etc. Recently, however, other types of lasers,.
The above energy level diagram shows the two excited states of helium atom, the 2 3S and 2 1S, that get populated as a result of the electromagnetic pumping in the discharge.
Both of these states are metastable and do not allow de-excitations via radiative transitions. Instead, the helium atoms give off their energy to neon atoms through collisional excitation.
In this way the 4s and 5s levels in neon get populated. These are the two upper lasing levels, each for a separate set of lasing transitions.
Radiative decay from the 5s to the 4s levels are forbidden. So, the 4p and 3p levels serve as the lower lasing levels and rapidly decay into the metastable 3s level.
In this way population inversion is easily achieved in the He-Ne. The 632.8 nm laser transition, for example, involves the 5s and 3p levels, as shown above.
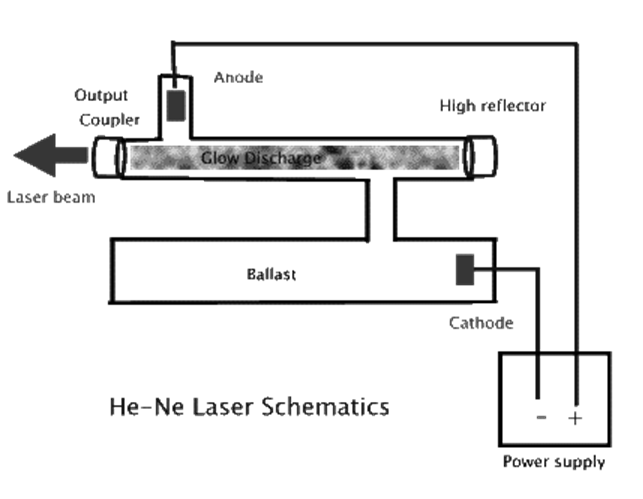
5.2.2 Gas Lasers Co2
The CO2 laser (carbon dioxide laser) is a molecular gas laser based on a gas mixture as the gain medium, which contains carbon dioxide (CO2), helium (He), nitrogen (N2), and probably some hydrogen (H2), water vapour, xenon (Xe). Such a laser is electrically pumped via an electrical gas discharge, which can be operated with DC current, with AC current (e.g. 20–50 kHz) or in the radio frequency (RF) domain. Nitrogen molecules are excited by the electric discharge into a metastable vibrational level and transfer their excitation energy to the CO2 molecules when colliding with them. The exited CO2 molecules then largely participate in the laser transition. Helium serves to depopulate the lower laser level and to remove the heat. Other constituents such as hydrogen or water vapor can help (particularly in sealed-tube lasers) to reoxidize carbon monoxide (formed in the discharge) to carbon dioxide.
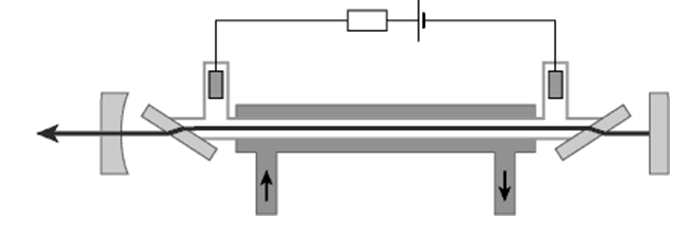
Figure 1: Schematic setup of a sealed-tube carbon dioxide laser. The gas tube has Brewster windows and is water-cooled.
CO2 lasers typically emit at a wavelength of 10.6 µm, but there are other spectral lines in the 9-11 µm region (particularly at 9.6 µm). In most cases, the average output powers are between a few tens of watts and many kilowatts. The power conversion efficiency can be much higher than 10%, i.e. it is higher than that of most gas lasers (due to a particularly favorable excitation path), even higher than that of pumped lamp solid-state lasers , but lower than many diodes. Pumped laser
Due to their high output power and long emission wavelengths, CO2 lasers require high-quality infrared optics, often made of materials such as zinc selenide (ZnSe) or zinc sulphide (ZnS ).
CO2 laser applications
CO2 lasers are widely used for the processing of laser materials, in particular for
• cut plastic materials, wood, matrix panels, etc., which have a high absorption at 10.6 μm and require moderate power levels of 20-200 W
• cut and weld metals such as stainless steel, aluminum or copper, applying powers of different kilowatts
• laser marking of various materials
Other applications include laser surgery (including ophthalmology) and scope research.
5.2.3Solid state laser, Dye Lasers:
A solid-state laser is a laser that uses the solid as the laser medium. Glass or crystalline materials are used in these lasers.
The ions are introduced as impurities in the host material, which can be glass or crystalline. The procedure of addition impurities to the substance is called doping. Rare earth elements such as cerium (Ce), erbium (Eu), terbium (Tb), etc. They are most commonly used as dopants.
Materials such as sapphire (Al2O3), neodymium doped aluminum, and yttrium garnet (Nd: YAG), neodymium doped glass (Nd: glass), and ytterbium doped glass are used as host materials for the laser medium.
Of these, neodymium doped yttrium-aluminum garnet (Nd: YAG) is most frequently used.
The first solid state laser was a ruby red laser. It is still used in some applications. In this laser, a ruby crystal is used as the laser medium.
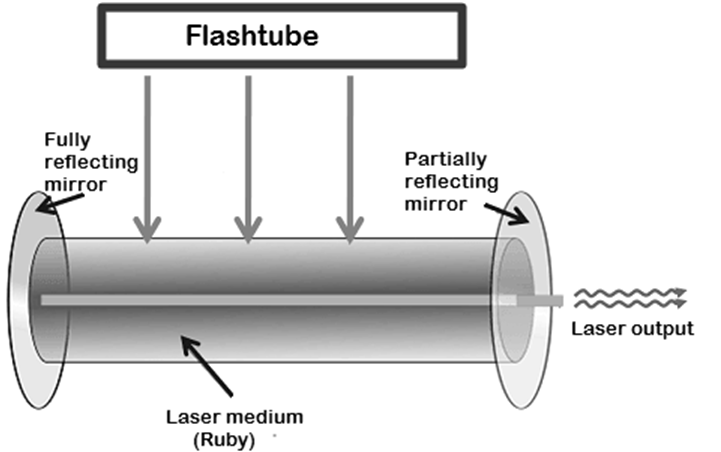
In solid state lasers, light energy is used as a pumping source. To achieve the pumping, light sources such as flash tubes, flash lamps, arc lamps or laser diodes are used.
Semiconductor lasers do not fall into this category because these lasers are generally electrically pumped and involve various physical processes.
A dye laser is a laser that uses an organic dye as a laser medium, usually as a liquid solution.
Compared to gases and most solid-state laser media, a dye can generally be used for a much wider range of wavelengths, often 50 to 100 nanometers or more.
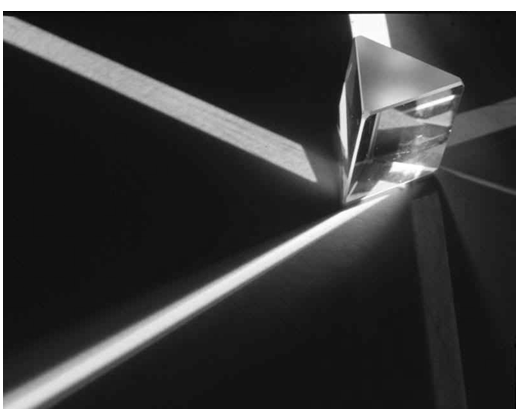
The light emitted by a laser is monochromatic, that is, it has a wavelength (color). In contrast, normal white light is a combination of different wavelengths (colors).
Directional Lasers emit highly directional light. Laser light is emitted as a comparatively narrow beam in a specific way.
Ordinary light, such as sunlight, a light bulb, or a candle, is emitted in many directions away from the source.
A laser light is said to be coherent, which means that the wavelengths of the laser light are in phase in space and time.
Monochromaticity. Laser light is monochrome means colored. So laser light is monochromatic light means that laser light is just a single color light. Monochromaticity is the main characteristic of the laser that makes it different from ordinary light.
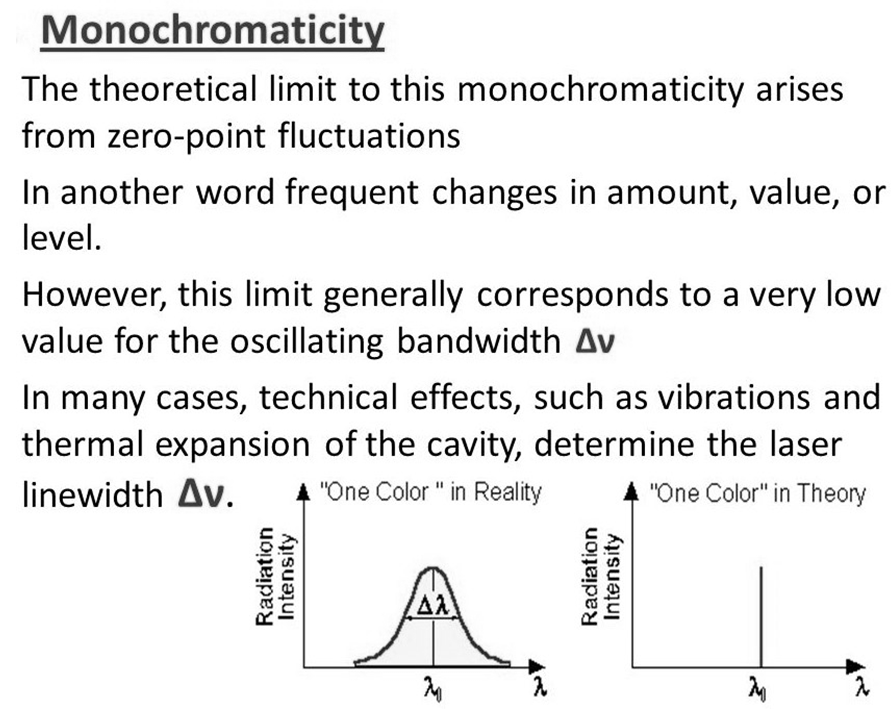
Reference:
1. G. MAIN, “VIBRATIONS AND WAVES IN PHYSICS”, CAMBRIDGE UNIVERSITY PRESS,1993.
2. H. J. PAIN, “THE PHYSICS OF VIBRATIONS AND WAVES”, WILEY, 2006.
3. E. HECHT, “OPTICS”, PEARSON EDUCATION, 2008.
4. A. GHATAK, “OPTICS”, MCGRAW HILL EDUCATION, 2012.
5. O. SVELTO, “PRINCIPLES OF LASERS”, SPRINGER SCIENCE & BUSINESS MEDIA, 2010.