Unit - 7
Chemical bonding in molecules
Atomic Orbitals is the mathematical function which is responsible for the determination of location and wave like behavior of an electron in the atom. Atomic orbital is the region where the electrons are present in the atoms. Hence the orbitals in an atom is identified from their unique values i.e.; n, l, m.
Properties:
- Electron orbits the nucleus as a standing waves.
- The electron is at the random position means they never stay at a single position.
Molecular Orbital is the mathematical function which is responsible for the determination of location and wave like behavior of an electron in the molecule. In molecular orbital, the electrons are allowed to interact with more than one atomic nucleus at a time.
Linear Combination of Atomic Orbital (LCAO):
The approximate method used to represent molecular orbital is called as the Linear Combination of Atomic Orbital. It is a quantum superposition of atomic orbital and a technique for calculating molecular orbital in quantum chemistry.
Rules for the Linear Combination of Atomic Orbital are:-
- The combining atoms should have the same symmetry along the molecular axis for proper combination e.g. All the sub-orbitals of 2p have same energy but still, the 2pz orbital of an atom can only combine with a 2pz orbital of another atom but cannot combine with 2px and 2py orbital as they have a different axis of symmetry.
- The two atomic orbital will combine to form molecular orbital. Greater is the extend of overlap of atomic orbital; greater will be the nuclear density.
The combining atomic orbital must be of equal energy or approximately same energy.
Bonding Molecular Orbitals
When addition of wave function takes place, the type of molecular orbitals formed are called Bonding Molecular orbitals and is represented by
ΨMO = ΨA + ΨB.
They have lower energy than atomic orbitals involved. It is similar to constructive interference occurring in phase because of which electron probability density increases resulting in formation of bonding orbital. Molecular orbital formed by addition of overlapping of two s orbitals. It is represented by s.
Anti-Bonding Molecular Orbitals
When molecular orbital is formed by subtraction of wave function, the type of molecular orbitals formed are called Antibonding Molecular Orbitals and is represented by
ΨMO = ΨA - ΨB.
They have higher energy than atomic orbitals. It is similar to destructive interference occurring out of phase resulting in formation of antibonding orbitals. Molecular Orbital formed by subtraction of overlapping of two s orbitals. It is represented by s* (*) is used to represent anti bonding molecular orbital) called Sigma Antibonding. Therefore, Combination of two atomic orbitals results in formation of two molecular orbitals, bonding molecular orbital (BMO) whereas other is anti-bonding molecular orbital (ABMO).
BMO has lower energy and hence greater stability than ABMO. First BMO are filled then ABMO starts filling because BMO has lower energy than that of ABMO.
Formation of molecular orbitals occurs by the combination of atomic orbitals of proportional symmetry and comparable energy. Therefore, a molecular orbital is polycentric and atomic orbital is monocentric. Number of molecular orbitals formed is equal to the number of atomic orbitals.
Certain rules are to be followed while filling up molecular orbitals with electrons in order to write correct molecular configurations:
Aufbau Principle – This principle states that those molecular orbital which have the lowest energy are filled first.
Pauli’s Exclusion Principle – According to this principle each molecular orbital can accommodate maximum of two electrons having opposite spins.
Hund’s Rule – This rule states that in two molecular orbitals of the same energy, the pairing of electrons will occur when each orbital of same energy consist one electron.
Energy Level Diagram:
The factors upon which relative energies of molecular orbitals depend are:
(i) Energies of the Atomic orbitals combining to form Molecular Orbitals.
(ii) The extent of overlapping between the atomic orbitals. The greater the overlap, the more the bonding orbital is lowered and the anti-bonding orbital is raised in energy relative to AOs
1s Atomic Orbitals (AOs) of two atoms form two Molecular Orbitals (MOs) designated as s1s and s *1s.The 2s and 2p orbitals (eight AOs of two atoms) form four bonding MOs and four anti-bonding MOs as:
Bonding MOs: σ 2s, σ 2pz, π 2px, π 2py
Anti – Bonding MOσ: σ *2s, σ *2pz, π *2px, π *2py
Using Spectroscopy, the energy levels of these molecular orbitals are determined experimentally. The order of increasing energy of molecular orbitals obtained by combination of 1s, 2s and 2p orbitals of two atoms is →
σ1s, σ *1s, σ 2s, σ *2s, σ 2pz, π 2px = π 2py, π *2px= π *2py, σ *2pz
MO Energy level Diagram for Homonuclear Diatomic molecules:
H2 molecule
H2 molecule consist of two H atoms and their two electrons. Two 1s orbitals give two MOs- one bonding that is σ and another one is antibonding that is σ*. The bonding orbital is in lower in energy state, the two electron occupies the bonding MO. The MO electron configuration of H2 molecule is written as (σ1s2).
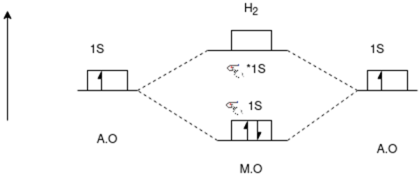
Energy evolved =
[No. Of electron in BMO (-) + No. Of electron in Anti Bonding Molecular Orbital * (+)]
=2*(- ) + 0*(+ )
= -2
This evolved energy is called as stabilization energy.
The molecule is stable so it is diamagnetic.
MO Energy level Diagram for Heteronuclear Diatomic molecules:
Carbon Monoxide Molecule (CO)
CO is the hetronuclear diatomic molecule. This molecule is formed by the combination of carbon and oxygen atom. Electronic configuration of carbon atom is 1s2, 2s2, 2p2 and that of oxygen is 1s2, 2s2, 2p4
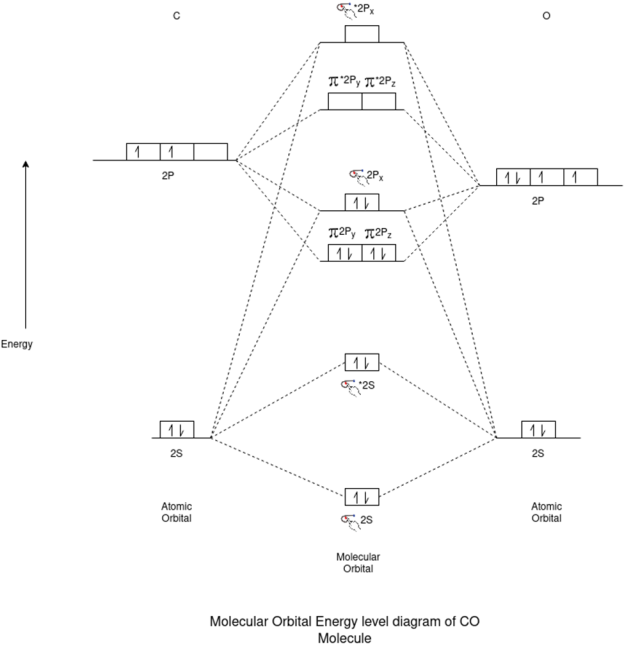
Stabilisation Energy
[No. Of electron in BMO * (-) + No. Of electrons in anti bonding molecular orbital * (+)]
=(8) * (-) + (2) * (+)
= -6
N2 Molecule:-
Electronic Configuration:-
N2 => σ1s2,σ*1s2,σ2s2,σ*2s2,π2px2,π2py2,π*2px2,π*2py2
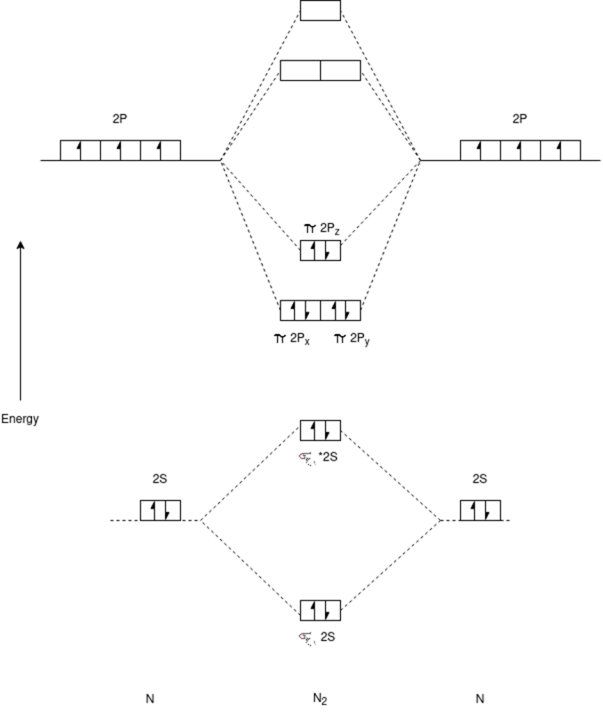
O2 Molecule:-
Electronic Configuration:-
N2 => σ1s2,σ*1s2,σ2s2,σ*2s2,π2px2,π2py2,π*2px1,π*2py1
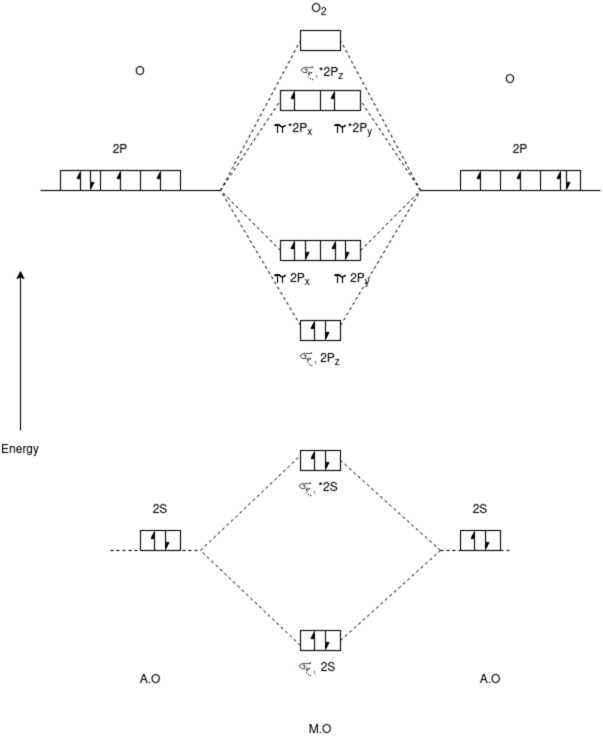
F2 Molecule:-
Electronic Configuration:-
F2=>σ1S2,σ*1S2,σ2S2,σ*2S2,π2P4,π*2P4

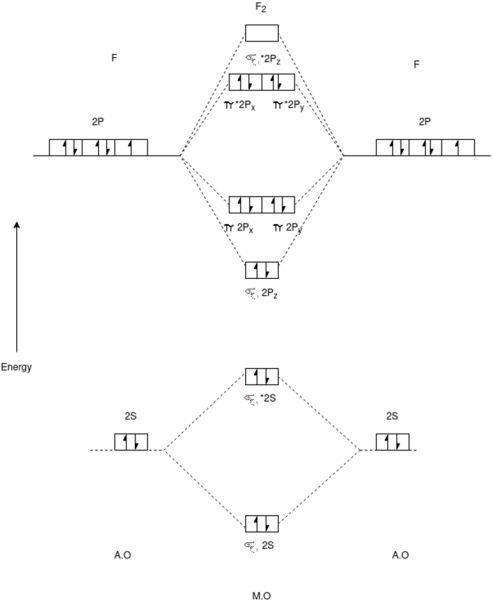
The chemical structures in which a central metal atom is surrounded by nonmetal atoms or groups of atoms, called ligands, joined to it by chemical bonds. Coordination compounds include such substances as vitamin B12, hemoglobin, and chlorophyll, dyes and pigments, and catalysts used in preparing organic substances. A major application of coordination compounds is their use as catalysts, which serve to alter the rate of chemical reactions. Certain complex metal catalysts, E.g.:, they play a key role in the production of polyethylene and polypropylene. In addition, a very stable class of organ metallic coordination compounds has provided impetus to the development of organo metallic chemistry. Organ metallic coordination compounds are sometimes characterized by “sandwich” structures, in which two molecules of an unsaturated cyclic hydrocarbon, which lacks one or more hydrogen atoms, bond on either side of a metal atom. This results in a highly stable aromatic system.
They possess the unusual chemical nature and useful properties of coordination compounds. The general class of coordination compounds or complexes, as they are sometimes called as extensive and diverse. The substances in the class may be composed of electrically neutral molecules or of positively or negatively charged species.
- A “coordination compound” is consisted of two components: the metal ion (atom) and the ligands.
- A ligand is an ion, a molecule, or a group that is associated with the metal ion as a Lewis acid-base adduct.
- The “donor atom” is the atom in the ligand, that attaches to the metal ion.
- A ligand has only one donor atom is a “monodentate”; with two donor atoms will be “bidentate”; “tridentate” for three; “tetradentate” for four…..etc.
- A ligand that has more than one donor atom is of “multi-dentate”.
- The association mold of a multidentate is called “chelation”.
- A “bridging ligand” attaches to more than one metal ion.
Nomenclature
Common Name | IUPAC Name | Formula | Structure |
Acetylacetonato | 2,4-pentanediono | CH3COCHCOCH3 | ![]() |
2,2-bipyridine | 2,2-bipyridine | Bipy | ![]() |
1,10-phenanthroline | 1,10-diaminophinanthrene | C12H8N2 | ![]()
|
Dialkyldithiocarbamato | Dialklylcarbamodithioato | S2CNR2 | ![]() |
Oxalate | Oxalate | C2O4 | ![]() |
Dimethylglyoximato | Butanediene dioxime | HONCC(CH3)C(CH3)NO | ![]() |
It is usual to study spectra in the range 200 nm (50 kK) to 1000 nm (10 kK), the near ultra-violet to the near infra-red. These energies correspond to electronic transitions between weakly bonding and weakly antibonding, bonding and non-bonding, and nonbonding and antibonding levels. At high energies almost all compounds are opaque. At lower energies, molecular vibrations complicate the spectra.
Spectra of d-d Transitions:
Many transition metal complexes show absorptions at relatively lower energies for electronic transitions (in the visible part of the spectrum). These are associated with transitions between d orbitals whose degeneracy has been removed by an environment of lower than spherical symmetry. E.g, in a octahedral complex, one may see transitions involving electron jumps from the t2g orbitals to the eg orbitals. These Orbitals are often only weakly bonding or antibonding - their energy separation is small. We may use the absorption energies to measure the ligand field splitting energies , but it should be noted that only in a few cases does the absorption energy correspond exactly to .
The study in which the transition metal complex ion absorbs electromagnetic radiation is called as the electron absorption spectroscopy. The absorbed radiation promotes electrons form the lower energy d-orbital to higher energy d-orbital. Such electronic transition are of high energy and in addition much lower energy vibrational and rotational transitions always occur. The vibrational and rotational levels are too close in energy. Therefore absorption bands due to high vibrational and rotational transitions are responsible for broadening of the electronic absorption in d-d spectra. Due to the promotion of electrons there occurs a change in the overall arrangement of electrons in d-orbital. The change in the overall arrangement of electrons in d-orbital gives rise to electron absorption spectrum.
The study of transition metal complex spectrum gives following:
(i) Color of complex
(ii) Magnitude of energy gap between ground state and excited state.
(iii) Extent of covalent character in metal ligand bond.
(iv) Geometry of the complex
(v) The position of metal ions and ligand in spectrochemical series and nephelauxetic series.
Atomic Spectroscopy: Atomic spectroscopy deals with the consequences of inter electronic repulsion and magnetic interactions between electrons of an atom or an ion. These interactions involve the coupling of a spin and angular momentum of electrons within a given electronic configuration. The coupling rises several degenerate and non degenerate energy levels these energy levels are known as the energy terms or spectroscopic states. The electronic transition in these energy levels gives rise to electronic absorption spectrum.
Splitting of degenerate energy terms: Electrostatic crystal field generated by ligands splits the degenerate energy terms of a given term electronic configuration of a metal ion in its complexes.
Selection Rules: Selection rules are used to determine allowed and forbidden transitions.
Measurement of electronic spectra of transition metal complexes:
The spectrum of a colored solution may be measured quite easily using a spectrophotometer. The beam of monochromatic light is passed through a solution and on to a photoelectric cell. The amount of light absorbed at any particular frequency can be read off or whole frequency range can be scanned. The absorbance If Io is the intensity of the original beam of light and I is the intensity after passing through the solution, then
A=log(Io/I)
The molar absorption coefficient is given by
E=A/Cl
Where,
C=concentration of solution
L= length
Organo metallic chemistry is the study of organo metallic compounds, chemical compounds containing at least one chemical bond between a carbon atom of an organic molecule and a metal, including alkaline, alkaline earth, and transition metals, and sometimes broadened to include metalloids like boron, silicon, and tin, as well. Aside from bonds to organyl fragments or molecules, bonds to 'inorganic' carbon, like carbon monoxide (metal carbonyls), cyanide, or carbide, are generally considered to be organometallic as well. Some related compounds such as transition metal hydrides and metal phosphine complexes are often included in discussions of organometallic compounds, though strictly speaking, they are not necessarily organometallic. The related but distinct term "metalorganic compound" refers to metal-containing compounds lacking direct metal-carbon bonds but which contain organic ligands. Metal β-diketonates, alkoxides, dialkylamides, and metal phosphine complexes are representative members of this class. The field of organometallic chemistry combines aspects of traditional inorganic and organic chemistry.
Organometallic (OM) chemistry is the study of compounds containing, and reactions involving, metal-carbon bonds. The metal-carbon bond may be transient or temporary, but if one exists during a reaction or in a compound of interest, we’re squarely in the domain of organometallic chemistry. Despite the denotation importance of the M-C bond, bonds between metals and the other common elements of organic chemistry also appear in OM chemistry: metal-nitrogen, metal-oxygen, metal-halogen, and even metal-hydrogen bonds all play a role. Metals cover a vast swath of the periodic table and include the alkali metals (group 1), alkali earth metals (group 2), transition metals (groups 3-12), the main group metals (groups 13-15, “under the stairs”), and the lanthanides and actinides. We will focus most prominently on the behavior of the transition metals, so called because they cover the transition between the electropositive group 2 elements and the more electron-rich main group elements.
