Unit 10
Lasers & Fibre Optics
Contents:
In order to have an insight into the principle of laser, we need to understand the way radiation field interacts with matter. In the early 20th century, Planck formulated the theory of spectral distribution of thermal radiation. Einstein, by combining Planck's theory and the Boltzmann statistics gave a theory of stimulated emission which is the governing principle of laser.
In thermal equilibrium at temperature T, with radiation frequency n and energy density u(v).
Let N1 and N2 be the number of atoms in energy states 1and 2 respectively at any instant. The numbers of atoms in state 1 absorb a photon and give rise to absorption per unit time
For equilibrium P12 = P21
N1 B12 u(v) = N2 [A21 + B21 u(v)]
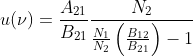
According to Boltzmann distribution law number of atoms N1 and N2 in energy states E1 and E2 in thermal equilibrium at temperature T are given by
Substituting N1/N2 in Eq, we get
Comparing it with Plank’s Radiation law
We get
(i)
B12 = B21, the probability of spontaneous emission is same as that of induced absorption. This means that if these two processes will occur at equal rates, so that no population inversion can be attained in a two-level system.
(ii)
The ratio of spontaneous emission and stimulated emission is proportional to v3. This implies that the probability of spontaneous emission dominates over induced emission more and more as the energy difference between the two states increases.
Population inversion
Under normal circumstances, the higher an energy level is, the less it is populated by thermal energy. Under some circumstances (for example, the presence of an upper energy level that has a relatively long lifetime), a system can be constructed so that there are more atoms/molecules in an upper energy level than is allowed under conditions of normal thermodynamic equilibrium. Such an arrangement is called a population inversion.
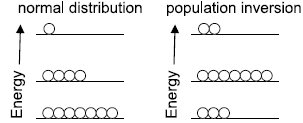
When a population inversion exists, an upper-state system will eventually give off a photon of the proper wavelength and drop to the ground state. This photon, however, can stimulate the production of other photons of exactly the same wavelength because of stimulated emission of radiation. Thus, many photons of the same wavelength (and phase, and other similar characteristics) can be generated in a short time. This is light amplification by stimulated emission of radiation, or LASER—usually seen in lowercase as laser. Lasers typically have a very narrow wavelength range of emission.
Many types of energy levels—electronic, vibrational, bands in semiconductors—are used to make lasers. Many materials are active laser sources, including Cr3+ ions in ruby and alexandrite crystals, Ti3+ in sapphire, Nd3+ in garnet, and a variety of doped semiconductors. Gases or gas mixtures like He/Ne, Ar, Kr, CO2, N2, even Cu(g) can be made to lase. Certain organic dyes can also be made to lase; one advantage is that their laser action has a broader range of wavelengths (20-30 nm) and they can be tuned to a particular wavelength.
Some imp. Points--
- Energy is applied to active medium raising active centres to excited energy level.
- These atoms spontaneously decay to a relatively long-lived, lower energy, metastable state
- A population inversion is achieved when the majority of atoms have reached this metastable state
- Lasing action occurs when an electron spontaneously returns to its ground state and produces a photon.
- If the energy from this photon is of the precise wavelength, it will stimulate the production of another photon of the
Same wavelength and resulting in a cascading effect.
6. The highly reflective mirror and partially reflective mirror continue the reaction by directing photons back through the medium along the long axis of the laser.
7. The partially reflective mirror allows the transmission of a small amount of coherent radiation that we observe as the “beam”.
8. Laser radiation will continue as long as energy is applied to the lasing medium.
Different types of lasers:
The most common and inexpensive gas laser, the helium-neon laser is usually constructed to operate in the red at 6328 Å. It can also be constructed to produce laser action in the green at 5435 Å and in the infrared at 15,230 Å.
The He – Ne laser has following main parts:
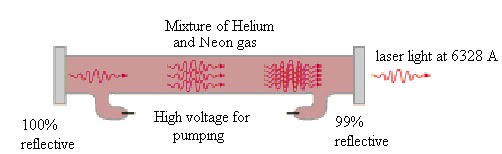
- The working substance (active medium)- is a long gas discharge tube filled with Helium and Neon gases in ratio 10:1. Ne atoms are active centres where He atoms helps Ne in achieving population inversion.
2. The resonance cavity- the two mirrors arranged externally one is fully reflective and other is partially transmissive forms the optical resonator.
3. Pumping scheme- atoms are excited by electrical discharge. The He atom is pumped to excited state by electrical discharge. One of the excited levels of helium at 20.61 eV is very close to a level in neon at 20.66 eV, so close in fact that upon collision of helium and a neon atom, the energy can be transferred from the helium to the neon atom during collision. Thus, the population inversion is achieved between E4 and E3 of Neon atom.
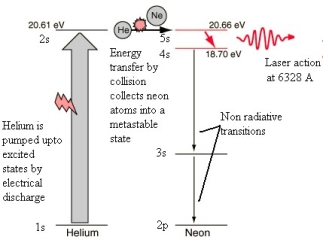
Ruby laser:
The ruby laser is the first type of laser actually constructed, first demonstrated in 1960 by T. H. Maiman. The ruby mineral (corundum) is aluminium oxide (Al2O3 ) with a small amount (about 0.05%) of chromium ions (Cr3+) which gives it its characteristic pink or red color by absorbing green and blue light.
The ruby laser is used as a pulsed laser, producing red light at 6943Å. After receiving a pumping flash from the flash tube, the laser light emerges for as long as the excited atoms persist in the ruby rod, which is typically about a millisecond.
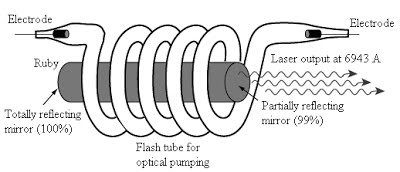
The ruby laser has following main parts:
- The working substance (active medium)- is in the form of a rod of ruby crystal (10 cm in length, 0.8 cm in diameter) in which Cr3+ are active centres while Al and O2 are inert.
- The resonance cavity- is made by silvering and polishing the ends of ruby rod. Fully reflecting plates at the left and a partially reflecting plate at the right, both optically plane and accurately parallel.
- The optical pumping system -consists of a helical xenon discharge tube. It produces flash of few milliseconds.
Ruby laser uses a three-level pumping scheme.
The xenon discharge generates a flash of white light for few milliseconds. The Cr+3 ions are excited to the E3 level by absorbing green and blue component of white light. From there the Cr+3 ions undergo non-radiative transitions and quickly drop to the metastable level E2. The metastable state has greater life time than E3. Therefore Cr+3 ions accumulate at E2. When more than half ions are accumulated at E2 the population inversion is established between E2 and E1. A chance spontaneous emission leads to chain stimulated emission. Red light (of wavelength 6943 Å) emerges from the front face.
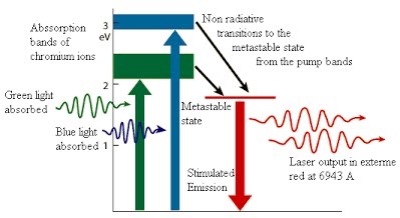
Neodymium-YAG Laser
It is a solid-state laser with four level laser system Neodymium-YAG is used to produce high power. Such lasers have been constructed to produce over a kilowatt of continuous laser power at 10.65 mm (in infra-red region) and can achieve extremely high powers in a pulsed mode.
The Nd: YAG laser has following main parts
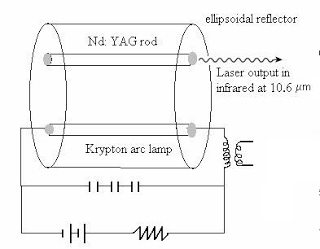
- The working substance (active medium)- is Yttrium-Aluminium-Garnet (Y3Al5O12), it is transparent and colourless. The neodymium- ion (Nd3+) replaces Y3+ ion in the yttrium-aluminium-garnet (YAG) which makes population inversion possible. Thus Nd3+ are active centres.
- 2 The resonance cavity- is made by silvering and polishing the ends of laser rod (Nd: YAG rod) to constitute the optical resonator. Fully reflecting plates at the left and a partially reflecting plate at the right, both optically plane and accurately parallel.
- The optical pumping system -The pumping if Nd3+ ion is done by krypton arc lamp. The light of wavelength 5000 – 8000 Å excites the ground state Nd3+ ions to the multiple energy levels at E4.
Efficiency of Nd:
YAG, laser is high in comparison to ruby laser which is about 2% (while for ruby laser it is only 1%).
It is a four-level system. The Nd3+ ions are excited to the pump bands (Level E4) by the white light (wavelength from 5000Å to 8000Å) of Argon arc lamp. From there they rapidly decay to metastable state E3. Soon the population inversion is established between E3 and E2. A spontaneous decay will start chain of stimulated emissions and laser light of wavelength 10.6 mm is emitted from the front. The Nd3+ ion reaches to the ground level in a non-radiative transition.
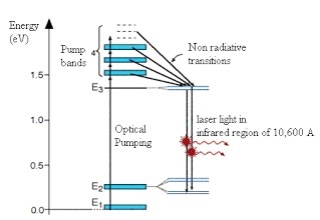
PN-junction Laser:
A semiconductor laser is a specially fabricated pn junction device (both the p and n regions are highly doped) which emits coherent light when it is forward biased. It is made from Gallium Arsenide (GaAs) which operated at low temperature and emits light in near IR region. Now the semiconductor laser is also made to emit light almost in the spectrum from UV to IR using different semiconductor materials. They are of very small size (0.1 mm long), efficient, portable and operate at low power. These are widely used in Optical fibre communications, in CD players, CD-ROM Drives, optical reading, laser printing etc.
p and n regions are made from same semiconductor material (GaAs). A p type region is formed on the n type by doping zinc atoms. The diode chip is about 500 mm long and 100mm wide and thick. The top and bottom faces has metal contacts to pass the current. The front and rare faces are polished to constitute the resonator (fig 1).
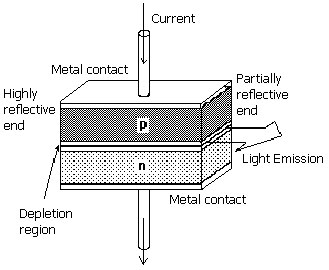
When high doped p and n regions are joined at the atomic level to form pn-junction, the equilibrium is attained only when the equalization of fermi level takes place in this case the fermi level is pushed inside the conduction band in n type and the level pushed inside the valence band in the p type (Fig 2).
When the junction is forward biased the at low voltage the electron and hole recombine and cause spontaneous emission. But when the forward voltage reaches a threshold value the carrier concentration rises to very high value. As a result, the region "d" contains large number of electrons in the conduction band and at the same time large number of holes in the valence band. Thus, the upper energy level have large number of electrons and the lower energy level has large number of vacancy, thus population inversion is achived. The recombination of elecrton and hole leads to spontaneous emmision and it stimulate the others to emit radiation. Ga As produces laser light of 9000 Å in IR region.
- Welding and Cutting: The highly collimated beam of a laser can be focused to a microscopic dot of extremely high energy density for welding and cutting. The automobile industry makes extensive use of carbon dioxide lasers with powers up to several kilowatts for computer-controlled welding on auto assembly lines.
- Communication: The lasers have significant advantages in communication because they are more nearly monochromatic and this allows the pulse shape to be maintained better over long distances when used in optical fibre.
- Barcode Scanner: Supermarket scanners typically use helium-neon lasers to scan the universal barcodes to identify products. The laser beam bounces off a rotating mirror and scans the code, sending a modulated beam to a light detector and then to a computer which has the product information stored.
- Laser Printing: The laser printer has in a few years become the dominant mode of printing in offices. The laser is focused and scanned across a photoactive selenium coated drum where it produces a charge pattern which mirrors the material to be printed. This drum then holds the particles of the toner to transfer to paper which is rolled over the drum in the presence of heat.
- CD’s and Optical Discs: The detection of the binary data stored in the form of pits on the compact disc is done with the use of a semiconductor laser.
- Surveying and Ranging: Helium-neon and semiconductor lasers have become standard parts of the field surveyor's equipment. A fast laser pulse is sent to a corner reflector at the point to be measured and the time of reflection is measured to get the distance.
- Laser cooling: The use of lasers to achieve extremely low temperatures has advanced to the point that temperatures of 10-9 K have been reached
- Laser Spectroscopy: Laser spectroscopy has led to advances in the precision with which spectral line frequencies can be measured, and this has fundamental significance for our understanding of basic atomic processes.
- Holography: Holography is "lens less photography" in which an image is captured not as an image focused on film, but as an interference pattern at the film.
Typically, coherent light from a laser is reflected from an object and combined at the film with light from a reference beam. This recorded interference pattern actually contains much more information that a focused image, and enables the viewer to view a true three-dimensional image which exhibits parallax. That is, the image will change its appearance if you look at it from a different angle, just as if you were looking at a real 3D object.
The optical fibre is a cylindrical, long, thin transparent structure made of glass and plastic, which is designed to guide the light wave from one end to another. The light inside the fibre is guided on the principle of Total Internal Reflection (TIR).
Optical fibers are widely used in fibre-optic communications to send information (data).
The basic structure of an optical fiber consists of three parts the core, the cladding, and the coating or buffer which are coaxially arranged. The innermost region is called the core, the light in the fibre travels only in the core. The core is surrounded by cladding, which is responsible for keeping the light inside the core. The refractive index of core (n1) is greater than of cladding (n2). The outermost region is called buffer or sheath, which protects the core and cladding from external abrasions
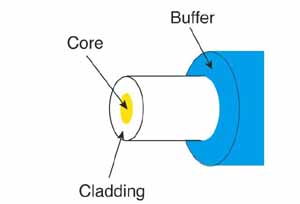
A) According to the number of modes
- Single Mode fibre (SMF): The core size of single mode fibers is small. The core size (diameter) is typically around 8 to 12 micrometres and only single mode (zero order) can travel through it.
- Multimode Fibre (MMF): The multimode fibers propagate more than one mode. The number of modes propagated depends on the core size and numerical aperture (NA). Typical values of fiber core size are 50 to 200 micrometre.
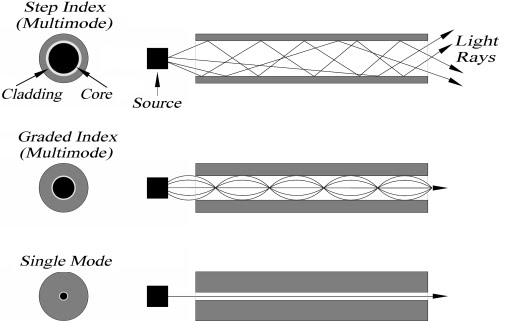
B) According to the Refractive index
- Step Index Fibre (SIF): In step index fibers, the refractive index of the core is uniform and undergoes an abrupt change at the core-cladding boundary
- Graded Index Fibre (GIF): In graded index fibers, the refractive index of the core varies gradually as a function of radial distance from the fiber center
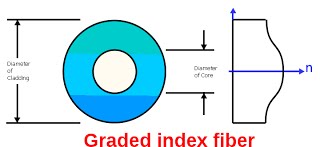
C) According to the Material
- Glass-Glass Fibre: The core and cladding are both made up of glass
- Plastic-Plastic Fibre: The core and cladding are both made up of plastic.
- PCS Fibre: Polyester Clad Silica Fibre (PCS) has silica core cladded with polyester.
A circular dielectric waveguide or fibre optic has an internal core that has a higher index of refraction than the cladding. At a certain diameter there is an angle that is less than the critical angle so there is total internal reflection.
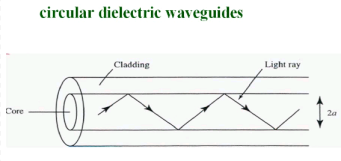
The phenomenon which occurs when the light rays travel from a more optically denser medium to a less optically denser medium.
Consider the following situation.
A ray of light passes from a medium of water to that of air. Light ray will be refracted at the junction separating the two media. Since it passes from a medium of a higher refractive index to that having a lower refractive index, the refracted light ray bends away from the normal. At a specific angle of incidence, the incident ray of light is refracted in such a way that it passes along the surface of the water. This particular angle of incidence is called the critical angle. Here the angle of refraction is 90 degrees. When the angle of incidence is greater than the critical angle, the incident ray is reflected back to the medium. We call this phenomenon total internal reflection.
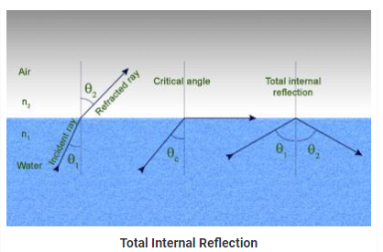

Notations Used in The Total Internal Reflection Formula and Critical Angle
- r is the angle of refraction
- i is the angle of incidence
- n1 is the refractive index in medium 1
- n2 is the refractive index in medium 2
- Ө is the critical angle
Following are the two conditions of total internal reflection:
- The light ray moves from a more denser medium to less dense medium.
- The angle of incidence must be greater than the critical angle.
When the incident ray falls on the cladding, it suffers total internal reflection as the angle formed by the ray is greater than the critical angle. Optical fibres have revolutionised the speed with which signals are transferred, not only across cities but across countries and continents making telecommunication one of the fastest modes of information transfer. Optical fibres are also used in endoscopy.
Numerical Aperture (NA)
The Numerical Aperture (NA) of a fibre is defined as the sine of the largest angle an incident ray can have for total internal reflectance in the core. Rays launched outside the angle specified by a fibber’s NA will excite radiation modes of the fiber. A higher core index, with respect to the cladding, means larger NA. However, increasing NA causes higher scattering loss from greater concentrations of dopant. A fiber's NA can be determined by measuring the divergence angle of the light cone it emits when all its modes are excited.
Qualitatively, NA is a measure of the light gathering ability of a fiber. It also indicates how easy it is to couple light into a fiber.
“V Number”
The Normalized Frequency Parameter of a fiber, also called the V number, is a useful specification. Many fiber parameters can be expressed in terms of V, such as: the number of modes at a given wavelength, mode cut off conditions, and propagation constants. For example, the number of guided modes in a step index multimode fiber is given by V2/2, and a step index fiber becomes single-mode for a given wavelength when V<2.405. Mathematically, V=2 π·NA·a/λ where “a” is the fiber core radius.
V – number determines how many modes a fiber can support, it is given by,

Where d is the diameter of the core, l is the wavelength of light used and NA is the numerical aperture of the fibre.
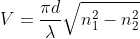
Or
If V ≤ 2.405, then the fibre is single mode fibre (SMF)
If V > 2.405, then the fibre is multimode fibre (MMF)
Number of Modes traveling in Fibre
The total number of modes traveling in a fibre depends on the V – Number and is related as:
For Step Index Fibre:

For Graded Index Fibre:
Attenuation is the loss of optical power as light travels along the fiber, caused by absorption, scattering, and bending losses. Signal attenuation is defined as the ratio of optical input power (Pi) to the optical output power (Po). The following equation defines signal attenuation as a unit of length:
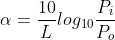
Factors of Attenuation
Absorption Loss
Absorption is caused by basic fiber-material properties as well as the impurity of transition metals and the presence of (OH-). Absorption in the ultraviolet region is caused by electronic absorption bands. The main cause of Absorption in the infrared region is the characteristic vibration frequency of atomic bonds.
If during fabrication transition metal impurities are introduced in the fiber material, such as iron, nickel, and chromium. Presence of (OH-) also increase absorption of light.
The amount of water (OH-) impurities present in a fiber should be less than a few parts per billion. Fiber attenuation caused by extrinsic absorption is affected by the level of impurities (OH-) present in the fiber. If the amount of impurities in a fiber is reduced, then fiber attenuation is reduced.
Scattering Loss
Scattering losses are caused by the interaction of light with density fluctuations within a fiber. Density changes are produced when optical fibers are manufactured. During manufacturing, regions of higher and lower molecular density areas, relative to the average density of the fiber, are created. Light traveling through the fiber interacts with the density areas and light is then partially scattered in all directions.
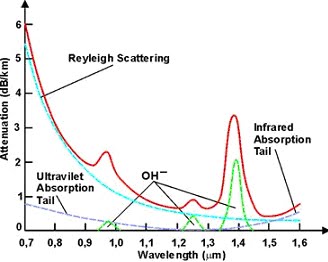
According to Rayleigh scattering loss is inversely proportional to the fourth power of the wavelength i.e., 1 / l4.
Microbend Loss
Microbend losses are small microscopic bends of the fiber axis that occur mainly when a fiber is cabled. These bends occur due to external forces, uneven coating applications and improper cabling procedures. Microbends change the path that propagating modes take, as shown in figure.
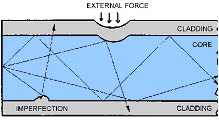
Macrobend Loss
Macrobend loss are bends having a large radius of curvature relative to the fiber diameter.
Mode Coupling Loss
Coupling loss in fiber optics refers to the power loss that occurs when coupling light from one optical device or medium to another
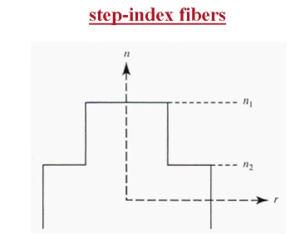
Step index fibre
These are referred to as step index fibers. The y axis refers to the index of refraction. The x axis is the distance from the centre of the core. So, the highest index of refraction (n1) is found in the core and there is a significant step in the index of refraction (n2) at the interface with the cladding.
In most practical waveguides the refractive indices of the core and cladding differ from each other by only a few percent.
The number of modes in a step-index circular waveguide is determined by the V parameter where
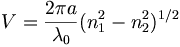
Where,
- Is the core radius
is referred to as the numerical aperture, the larger this term the larger the number of modes.
The fibre can support only one mode when V < 2.405
For n1 = 1.48 and n2 = 1.46, the radius of the core a <2.7μm
Graded index fibre
There is current research to develop a core with a graded index of refraction that gradually decreases to equal that of the cladding. This is referred to as a graded index fibre.
- Medical
Used as light guides, imaging tools and also as lasers for surgeries - Defence/Government
Used as hydrophones for seismic waves and SONAR , as wiring in aircraft, submarines and other vehicles and also for field networking - Data Storage
Used for data transmission - Telecommunications
Fiber is laid and used for transmitting and receiving purposes - Networking
Used to connect users and servers in a variety of network settings and help increase the speed and accuracy of data transmission - Industrial/Commercial
Used for imaging in hard to reach areas, as wiring where EMI is an issue, as sensory devices to make temperature, pressure and other measurements, and as wiring in automobiles and in industrial settings - Broadcast/CATV
Broadcast/cable companies are using fiber optic cables for wiring CATV, HDTV, internet, video on-demand and other applications
Fiber optic cables are used for lighting and imaging and as sensors to measure and monitor a vast array of variables. Fiber optic cables are also used in research and development and testing across all the above-mentioned industries.