Unit 1
Introduction
Content:
Basics of prime mover
In earlier days when there were no technological advancement or no inventions in the field of development of the mechanical machines, human being had to depend on his own physical strength and on animal for various works to carry out. But Now with the development of engines and many energy sources to produce power from the engines, it became possible to meet different needs in our day to day life and working.
Definition of prime mover:
Prime mover is device, which use the energy from natural sources and convert it into the mechanical energy.
Various sources of energy used by prime movers:
Prime movers use the different sources of energy. The various sources are as follow:
Burning of fuels:
This is a most important source of energy. When any fuel is burnt it releases the heat energy. The energy given out by fuel depends on the type of the fuel burnt. Different fuels have different calorific values and based on that heat is liberated by fuel. Then this heat energy is converted into the mechanical energy.
River water: It is another useful and widely used source of energy. Water flowing from certain height contains the potential energy. This potential energy is converted into kinetic energy when it reaches to the prime mover. Here prime mover is hydraulic turbine through which power is generated. The use of river water is to produce steam in a boiler. Again, this steam is used in steam turbines and steam engines to produce the mechanical power.
Atoms: Now days with recent development atoms are used as a good and rich source of energy. Heat energy is produced by fission or fusion of atoms. This heat energy is converted into mechanical work by engines
.
Wind Energy: This source of energy is widely used now a days because it is available freely everywhere. It is a renewable source of energy.
Solar energy: The source of energy is also freely available so it is trapped to make its proper use to generate power .It is also falling in the category of renewable source of energy.
Types of prime movers:
The prime movers are classified based on the sources of energy utilized by them. The classification is shown below:
1)Thermal prime movers: These are the prime movers which use the thermal energy of source to generate power. Various thermal prime movers are given below:
Fuels (heat engines): These prime movers use various fuels like petrol, diesel, oil, gas to generate mechanical power.
Heat engines are two types:
-External combustion engines:
1)Reciprocating steam engines
2)Steam turbine
3)Closed cycle gas turbine
-Internal combustion engines:
1)Reciprocating I.C. Engines
2)Open cycle gas turbine
Nuclear(nuclear power plant): This prime mover uses the heat energy of atoms by fission or fusion process to develop the mechanical power. It is mainly used in nuclear power plants. Various radioactive elements like uranium, thorium are used for these fission or fusion process in a nuclear reactor.
Geothermal: In this type of prime mover the heat energy is obtained from a certain depth or the hot part of the earth below earth surface then it is converted into mechanical by proper engine.
Bio gas: Bio gas is mainly produced from a garbage or any other waste which is used to produce power by prime mover in a biogas plant.
Solar energy: The solar energy come to the earth in the form of radiation or electromagnetic waves. This energy trapped in with the help of solar panel made up of semiconductor material. This heat energy is then converted into power.
2) Non-thermal prime movers: These kinds of prime movers do not use the heat energy to convert it into the mechanical power. The following are the non-thermal prime movers:
Hydraulic turbines: This type of the prime mover uses the stored potential energy of water to generate power.
Wind power: With the help of wind turbine the wind energy is converted into the power.
Tidal power: The energy of tides from ocean is converted into the power by the use of turbine which is known as tidal power.
Push or pull of an object is considered a force. Push and pull come from the objects interacting with one another. Terms like stretch and squeeze can also be used to denote force.
In Physics, force is defined as: The push or pull on an object with mass that causes it to change its velocity.
Force is an external agent capable of changing the state of rest or motion of a particular body. It has a magnitude and a direction. The direction towards which the force is applied is known as the direction of the force and the application of force is the point where force is applied.
The Force can be measured using a spring balance. The SI unit of force is Newton (N).
Common symbols: F→, F
SI unit: Newton
In SI base units: kg.m/s2
Other units: dyne, pound, pound-force, kip, kilo pond
Derivations from other quantities: F = m a
Dimension: LMT-2
Effects of Force
In physics, motion is defined as the change in position with respect to time. In simpler words, motion refers to the movement of a body. Typically, motion can either be described as:
- Change in speed
- Change in direction
- The Force has different effects and here are some of them.
- Force can make a body that is at rest to move.
- It can stop a moving body or slow it down.
- It can accelerate the speed of a moving body.
- It can also change the direction of a moving body along with its shape and size.
Formula for Force
The quantity of force is expressed by the vector product of mass (m) and acceleration (a). The equation or the formula for force can mathematically be expressed in the form of:
F = ma
Where,
M = mass
A = acceleration
It is articulated in Newton (N) or Kgm/s2.
Acceleration a is given by
A = v/t
Where
V = velocity
T = time taken
So, Force can be articulated as:
F = mv/t
Inertia formula is termed as p = mv which can also be articulated as Momentum.
Therefore, Force can be articulated as the rate of change of momentum.
F = p/t = dp/dt
Force formulas are beneficial in finding out the force, mass, acceleration, momentum, velocity in any given problem.
Unit of Force
In the centimeter gram second system of unit (CGS unit) force is expressed in dyne. In the standard international system of unit (SI unit) it is expressed in Newton (N).
Types of Force
Force is a physical cause that can change the state of motion or the dimensions of an object. There are two types of forces based on their applications:
Contact Force
Non-Contact Force
Contact Force
Contact Force
Forces that act on a body either directly or through a medium are called contact forces.
Examples of contact forces are:
Muscular Force
Mechanical Force
Frictional Force
We can make use of the muscular force of animals like bullocks, horses, and camels to get the activities done. The frictional force is another type of contact force, which acts between a pair of a surface in contact and tends to oppose the motion of one surface over the other.
Non-Contact Force: Forces that act through spaces without making direct contact with the body are called non-contact forces.
Examples of non-contact forces are:
Gravitational Force
Electrostatic Force
Magnetic Force
The force exerted by a magnet on other magnets is called magnetic force. Magnetic force and electrostatic force act on an object from a distance, that’s the reason they are non-contact forces. The strength of gravity is an attractive force that is exerted by the Earth on objects, which make them fall to the land. The weight of a body is the force that is pulled by the earth towards the center.
The amount of force exerted (thrust) on a surface per unit area is defined as ‘Pressure’. It can also be defined as the ratio of the force to the area (over which the force is acting).
Formula and Unit of Pressure
Pressure (P) = Thrust/ Area
The SI unit is ‘Pascals (Pa)’. 1 Pa = 1N/m2
Example: It is easier to hammer a sharp pin than to hammer a blunt pin. This is because the area at the end of the sharp pin is smaller than the area at the end of a blunt pin. This leads to an increase in pressure leading to hammer the sharp pin easily.
Types of Pressure
Atmospheric Pressure
Absolute Pressure
Differential Pressure
Gauge Pressure
Atmospheric Pressure
The earth’s atmospheric air is surrounded by a layer of gases and so this air surrounding the earth exerts a pressure known as the ‘atmospheric pressure’. Its value at sea level is 101325 Pa.
It is measured using a mercury barometer (hence atmospheric pressure is also known as barometric pressure), indicating the height of a column of mercury which exactly balances the weight of the column of atmosphere over the barometer. It can be expressed in several different systems of units such as millimeters (or inches) of mercury, pounds per square inch (psi), dynes per square centimeter, millibars (mb), standard atmospheres, or kilopascals.
The atmospheric pressure decreases near Earth’s surface, with height at a rate of about 3.5 millibars for every 30 meters (100 feet).
Energy is essential to life and all living organisms. The sun, directly or indirectly, is the source of all the energy available on Earth. In Physics, energy is a quantitative property that must be transferred to an object in order for it to perform work. Hence, we can define energy as the strength to do any kind of physical activity. Thus, they say,
Energy is the ability to do work
Energy is a conserved quantity and the law of conservation of energy states that energy can neither be created nor destroyed but can only be converted from one form to another. The SI unit of energy is Joule.
Units of Energy
The International System of Units of measurement of energy is Joule. The unit of energy is named after James Prescott Joule. Joule is a derived unit and it is equal to the energy expended in applying a force of one newton through a distance of one meter. However, energy is also expressed in many other units not part of the SI, such as ergs, calories, British Thermal Units, kilowatt-hours, and kilocalories, which require a conversion factor when expressed in SI units.
Energy Conversion: Transfer and Transform the movement of energy from one location to another is known as energy transfer. There are energy transfers going on all the time. Whenever a system changes there is a change in the way some or all of the energy is stored.
Following are the four ways through which energy can be transferred:
Mechanically – By the action of force electrically
Electrically by Radiation – By Light waves or Sound waves By Heating – By conduction, convection, or radiation the process which results in the energy changing from one form to another is known as energy transformation. While energy can be transformed or transferred, the total amount of energy does not change – this is called energy conservation. Read More: Energy Conversion Law of Conservation of Energy The law of conservation of energy is one of the basic laws in physics. It governs the microscopic motion of individual atoms in a chemical reaction. The law of conservation of energy states that “In a closed system, i.e., a system that is isolated from its surroundings, the total energy of the system is conserved.” According to the law, the total energy in a system is conserved even though the transformation of energy occurs. Energy can neither be created nor destroyed; it can only be converted from one form to another.
Different Types of Energy
Forms of Energy
Although there are many forms of energy, it is broadly categorized into kinetic energy and potential energy.
Kinetic Energy
Kinetic energy is the energy associated with the object’s motion. Objects in motion are capable of causing a change or are capable of doing work. To better understand, let us think of a wrecking ball. A wrecking ball in motion is used to do work such as demolition of buildings, stones, etc. Even a slow-moving wrecking ball is capable of causing a lot of damage to another object such as an empty house. However, a wrecking ball that is not in motion, does not do any work.
Another example of kinetic energy is the energy associated with the constant, random bouncing of atoms or molecules. This is also known as thermal energy. The average thermal energy of a group of molecules is what we call temperature, and when thermal energy is being transferred between two objects, it’s known as heat.
Kinetic energy is determined by the given formula K.E=1/2mv2
Different types of Kinetic Energy include:
Radiant energy
Radiant energy refers to the type of energy that travels by waves or particles. This energy is created through electromagnetic waves and is most commonly experienced by humans in the form of heat. Following are a few examples of radiant energy:
When you turn on an incandescent light bulb, it gives off two forms of energy. There is visible light and heat that is generated. Both these generated energies are a form of radiant energy.
Sunlight is an example of radiant energy.
Thermal Energy
Thermal energy is similar to radiant energy and is experienced in the form of heat or warmth. While radiant energy refers to waves or particles, thermal energy describes the level of activity among the atoms and molecules in an object. This is the only difference between radiant energy and thermal energy. Some examples of thermal energy include:
The geothermal energy that comes from the decay of natural minerals and the volcanic action of the earth is an example of thermal energy.
When you heat up the pizza in the oven, you are raising the temperature of the pizza. The molecules that make up the pizza are moving more quickly when the pizza is piping hot.
The warmth that you feel emanating from the engine is an example of thermal energy.
Sound Energy
The vibrations that reach the human ear are experienced by humans as sound. The disturbance moves in the form of waves through a medium like air and reaches our eardrum. On reaching the eardrum, these vibrations are converted into electrical signals and sent to the brain which we interpret as the sensation of sound.
Electrical Energy
The flow of negatively charged electrons around a circuit results in electricity which we more commonly referred to as electrical energy.
Mechanical Energy
Mechanical energy is the energy associated with the mechanical movement of objects.
Potential Energy
Potential energy is the energy stored in an object or system of objects. Potential energy has the ability to transform into a more obvious form of kinetic energy.
Potential energy is determined by the given formula
Potential Energy= m×g×h
Both potential energy and kinetic energy form mechanical energy.
Mechanical energy is determined by the following formula
Mechanical Energy=1/2mv2+mgh
Different types of potential energy include
Gravitational Potential Energy Gravitational potential energy is the energy stored in an object as the result of its vertical position or height. A book on a high bookshelf has a higher gravitational potential energy than the book at the bottom bookshelf.
Elastic Potential Energy
Elastic potential energy is energy stored as a result of applying a force to deform an elastic object. The energy is stored until the force is removed and the object springs back to its original shape, doing work in the process. The deformation could involve compressing, stretching or twisting the object.
Chemical Potential Energy
Chemical potential energy is the energy stored in the chemical bonds of the substance. It is the energy that can be absorbed and released due to a change in the particle number of the given species.
Electric Potential Energy
Electric potential energy is the energy that is needed to move a charge against an electric field.
Some of the examples of electric potential energy include:
An incandescent light bulb that is turned off
A radio tower that is not working
A black-light turned off
A television before it is turned on
The scientific definition of work is different in many ways from its everyday meaning. The definition of work in physics reveals its relationship to energy – whenever work is done, energy is transferred.
For a work to be done, in a scientific sense, a force must be exerted and there must be displacement in the direction of the force. With this said, we can say that
Work is the product of the component of the force in the direction of the displacement and the magnitude of this displacement.
Mathematically, the above statement is expressed as follows:
W = (F cos θ) d = F. d
Where,
W is the work done by the force.
F is the force; d is the displacement caused by the force
Θ is the angle between the force vector and the displacement vector
The dimension of work is the same as that of energy and is given as = [ML2T–2].
Unit of Work
The SI unit of work is the joule (J), which is defined as the work done by a force of 1 Newton in moving an object through a distance of 1 meter in the direction of the force.
Work
A weightlifter lifts a barbell weighing 25 kg and displaces it from the ground by 2 m. Here, the work done upon the barbell is against gravity.
The work done upon the weight against gravity can be calculated as follows:
Work done = (Mass × acceleration due to gravity) × Displacement
= (25 × 9.8) × 2 J
We can define power as the rate of doing work, it is the work done in unit time. The SI unit of power is Watt (W) which is joules per second (J/s). Sometimes the power of motor vehicles and other machines is given in terms of Horsepower (hp), which is approximately equal to 745.7 watts.
Average Power
We can define average power as the total energy consumed divided by the total time taken. In simple language, we can say that average power is the average amount of work done or energy converted per unit of time.
Power Formula
Power is defined as the rate at which work is done upon an object. Power is a time-based quantity. This is related to how fast a job is done. The formula for power is mentioned below.
Power = Work / time
P = W / t
Unit of Power
The unit for standard metric work is the Joule and the standard metric unit for time is the second, so the standard metric unit for power is a Joule / second, defined as a Watt and abbreviated W.
A system, as it is defined in physics or chemistry, is nothing more than a collection of objects (or smaller systems) that can be identified. Usually, the word system refers to a collection that makes thinking about a problem more convenient. The surrounding is everything else that is not the system defined. For example, if the system being studied is a house, the surrounding would be everything else that is not the house (other houses, the neighbourhood, the general environment around the house, etc.).
Systems can be described in three different ways.
Isolated: this is a system in which no matter or energy is being exchanged with the surroundings.
Closed: this is a system in which only energy is being exchanged with the surroundings.
Open: this is a system in which both matter and energy is being exchanged with the surroundings. Often, the most convenient system is an isolated system, one where outside influences can be ignored (either because they cancel out or because outside influences are negligible). For example, two balls rolling across a smooth surface to strike each other can be considered an isolated system, while two balls rolling across a gravel path or thick carpet are influenced by friction originating from outside the system and therefore are not an isolated system.
While anything can be a system, much of the study of physics and chemistry is about figuring out which systems are useful to consider in studying a particular property or relationship of items within the system.
Heat is the transfer of kinetic energy from one medium or object to another or from an energy source to a medium or object. Such energy transfer can occur in three ways: radiation, conduction, and convection.
The standard unit of heat in the International System of Units (SI) is the calorie (cal), which is the amount of energy transfer required to raise the temperature of one gram of pure liquid water by one degree Celsius, provided the water temperature is higher than the freezing point and lower than the boiling point. Sometimes the kilocalorie (kcal) is specified as a unit of heat; 1 kcal = 1000 cal. (This is the so-called diet calorie.) Less often, the British thermal unit (Btu) is used. This is the amount of heat required to raise the temperature of one pound of pure liquid water by one degree Fahrenheit.
An example of heat by radiation is the effect of infrared (IR) energy as it strikes a surface. IR is an electromagnetic field capable of transferring energy from a source, such as a fireplace, to a destination, such as the surfaces within a room. Radiation does not require an intervening medium; it can occur through a vacuum. It is responsible for the warming of the Earth by the sun.
Heat by conduction takes place when two material media or objects are in direct contact, and the temperature of one is higher than the temperature of the other. The temperatures tend to equalize; thus, the heat conduction consists of a transfer of kinetic energy from the warmer medium to the cooler one. An example is the immersion of a chilled human body in a hot bath.
Heat by convection occurs when the motion of a liquid or gas carries energy from a warmer region to a cooler region. A good example of convection is the tendency of warm air to rise and cool air to fall, equalizing the air temperature inside a room containing a hot stove. Heat convection (along with conduction) is believed to take place inside the Earth, transferring kinetic energy from the inner core through the outer core and mantle to the crust. In this situation, the outer core and the mantle behave like liquids over long periods of time.
Temperature is the degree or intensity of the heat present in a substance or a system, expressed based on the comparative scale and shown by a thermometer.
In other words, Temperature is the measure of hotness or coldness of a body measured using Celsius, Kelvin, and Fahrenheit scales.
The change in temperature is based on the amount of heat released or absorbed. The S.I unit of temperature is Kelvin.
The Temperature formula is given by,
Δ T = Q / mc
Where,
Δ T = temperature difference,
Q = amount of heat absorbed or released,
m = mass of the body,
c = specific heat of the body.
Specific heat capacity is the amount of heat energy required to raise the temperature of a substance per unit of mass. The specific heat capacity of a material is a physical property. It is also an example of an extensive property since its value is proportional to the size of the system being examined.
In SI units, specific heat capacity (symbol: c) is the amount of heat in joules required to raise 1 gram of a substance 1 Kelvin. It may also be expressed as J/kg•K. Specific heat capacity may be reported in the units of calories per gram degree Celsius, too. Related values are molar heat capacity, expressed in J/mol•K, and volumetric heat capacity, given in J/m3•K. Heat capacity is defined as the ratio of the amount of energy transferred to a material and the change in temperature that is produced:
C = Q / ΔT
Where C is heat capacity, Q is energy (usually expressed in joules), and ΔT is the change in temperature (usually in degrees Celsius or in Kelvin).
State:
The application of thermodynamic principles begins by defining a system that is in some sense distinct from its surroundings. For example, the system could be a sample of gas inside a cylinder with a movable piston, an entire steam engine, a marathon runner, the planet Earth, a neutron star, a black hole, or even the entire universe. In general, systems are free to exchange heat, work, and other forms of energy with their surroundings.
A system’s condition at any given time is called its thermodynamic state. For a gas in a cylinder with a movable piston, the state of the system is identified by the temperature, pressure, and volume of the gas. These properties are characteristic parameters that have definite values at each state and are independent of the way in which the system arrived at that state. In other words, any change in value of a property depends only on the initial and final states of the system, not on the path followed by the system from one state to another. Such properties are called state functions. In contrast, the work done as the piston moves and the gas expands and the heat the gas absorbs from its surroundings depends on the detailed way in which the expansion occurs.
The behaviour of a complex thermodynamic system, such as Earth’s atmosphere, can be understood by first applying the principles of states and properties to its component part in this case, water, water vapour, and the various gases making up the atmosphere. By isolating samples of material whose states and properties can be controlled and manipulated, properties and their interrelations can be studied as the system changes from state to state.
Path: If all the change of states of system are plotted and all the points are conned, then the line joining the change of states of the system is called the path
Process:
Thermodynamics process represents a transition in which a system changes from one state to another. When the path is completely specified then the change of state is called a process. A Process is defined as the transformation of the system from one fixed state to another fixed state .When any one of the properties changes, the working substance or system is said to have undergone a process.
Some of the processes are identified by special names as given below:
- Isobaric process (constant pressure process)
- Isochoric process (constant volume process)
- Isothermal process (constant temperature process)
- Isentropic process (constant entropy process)
- Adiabatic process(perfectly insulated process)
Enthalpy:
In thermodynamics process constant enthalpy means that enthalpy does not change in all process. Best understand the concept of constant enthalpy we should understand the throttling process.
Throttling Process:
The throttling process is an expansion process in which the pressure reduces after expansion and the velocity is negligible. A throttling expansion occurs when a gas or a vapour is expanded through an aperture of minute dimensions such as a throat or a slightly opened valve.
During the throttling process the expanding fluid is forced through the aperture by its pressure but the hole is so narrow that the frictional resistance between the fluid and the wall reduces the fluid velocity to a negligible amount; as a result, the fluid escapes with a small amount of kinetic energy.
Due to friction kinetic energy reappears as heat and the gas is raised to its initial temperature. During a throttling process no heat is supplied or rejected, no external work is done and in the case of a perfect gas there is no alteration in temperature.
When a fluid expands through a throttle valve or a constricted orifice, the enthalpy before the throttling valve is equal to the enthalpy after throttling. This does not mean, however, that the throttling process is constant enthalpy process. Here the properties before and after the process are defined. Fig. 2-24 shows throttling process.
H1 = H2.
In this process the enthalpy remains constant. The enthalpy at condition 1 is equal to enthalpy at condition 2.
Internal Energy:
As usual, we get change in internal energy = U2 – U1 = m x Cv (T2 – T1).
Another way of determination of change in internal energy is very common in isentropic operation.
By first law of thermodynamics as applied to non-flow process,
Heat supplied = change in internal energy + work done; but heat supplied is zero
Change in internal energy = – work done.
Thus, we get an important relation in an isentropic process. This relation can be stated as “Change in internal energy is numerically equal to work done”. When the work is done by the gas, it loses internal energy and it gains internal energy when the work is done on the gas.
Cycle: When a system goes through different change of states and return backs to the original state, i.e., all the properties are identical to the original state, then the system is said have gone through a thermodynamic cycle.
Zeroth Law of thermodynamics
If two systems are each in thermal equilibrium with the third system then the two systems are in thermal equilibrium which each other.
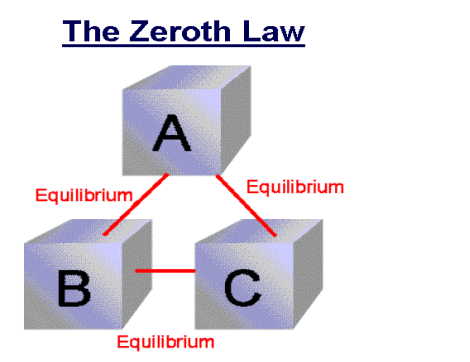
When a body A is in thermal equilibrium with a body B , and also separately with a body C ,then B and C will thermal equilibrium with each other .this is known as the Zeroth law of thermodynamics.
First law of Thermodynamics
Sometimes our body start to sweat and feel warm when we are in a room full of people and the sweating becomes excessive if the room size is small. This happens because our body is trying to cool off hence heat transfers from our body in the form of ‘sweat’. This entails the first law of thermodynamics.
The first law of thermodynamics states that the total energy of an isolated system is constant. Energy can be transformed from one form to another, but can neither be created nor destroyed.
According to this law, some of the heat given to system is used to change the internal energy while the rest in doing work by the system. Mathematically,
ΔQ=ΔU+ΔW
Where,
ΔQ = Heat supplied to the system
ΔW= Work done by the system.
ΔU = Change in the internal energy of the system.
If Q is positive, then there is a net heat transfer into the system, if W is positive, then there is work done by the system. So positive Q adds energy to the system and positive W takes energy from the system.
It can also be represented as ΔU=ΔQ−W
We can say that internal energy tends to increase when heat is given to the system and vice versa.
Limitations of First Law of Thermodynamics
- The limitation of the first law of thermodynamics is that it does not say anything about the direction of flow of heat.
- It does not say anything whether the process is a spontaneous process or not.
- The reverse process is not possible. In actual practice, the heat doesn’t convert completely into work. If it would have been possible to convert the whole heat into work, then we could drive ships across the ocean by extracting heat from the water of the ocean.