Unit 4
Stereochemistry, Reaction Mechanism, and synthesis of drug molecules
A three-dimensional (3-D) structure of organic molecules can be represented on paper by using certain conventions. A solid () wedge is used to indicate a bond projecting out of the plane of the paper, towards the observer. A dashed () wedge is used to indicate a bond projecting out of the plane of the paper, away from the observer.
Fig 1: An example of a 3D representation of organic molecules
Isomerism
Two or more compounds having the same molecular formula but different physical and chemical properties are called isomers and the phenomenon is known as isomerism. There are two types of isomerism
- Structural isomerism.
- Stereoisomerism.
Structural isomerism
Those compounds that have the same molecular formula but have different structures are called structural isomers. Structural isomerism is further divided into six subcategories.
- Chain isomerism
The compounds that have the same molecular formula but differ in carbon skeletons are called chain isomers and the phenomenon is known as Chain isomerism.
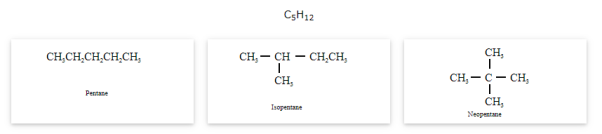
Position isomerism
The compounds that have the same molecular formula but are different with respect to the position of the multiple bonds (double or triple bond) or functional group are called position isomers and the phenomenon is known as position isomerism.
Functional isomerism
The compounds that have the same molecular formula but differ in their functional groups are called functional isomers and the phenomenon is called functional isomerism.

The general formula for ether is R-O-R' where R and R' are different (or similar) carbon chains.
Metamerism
The compounds that have the same formula but differ in alkyl chains on either side of the functional group are called metamers and the phenomenon is known as metamerism.

Notice that the above compounds are also called position isomers.
Tautomerism
Tautomerism occurs due to the 1,3-migration of a hydrogen atom within the same molecule. The best example of tautomerism is keto-enol tautomerism, where one form contains the keto group (>C=O) and the other form contains the enol (en + ol) group.
Acetaldehyde and ethanol

You may note that in keto-enol tautomerism, enol form is usually negligible.
Ring-chain isomerism
The compounds that have the same molecular formula but one possesses an open-chain while the others possess cyclic structures are called ring-chain isomers and the phenomenon is known as ring-chain isomerism.
Stereoisomerism
The compounds that have the same formula but are different in the spatial arrangement of one atom or group of atoms in space are known as stereoisomers and the phenomenon is called stereoisomerism. Stereoisomerism has the following two subcategories:
- Conformational isomerism
- Configurational isomerism
- Conformational Isomerism
Conformational isomers are those isomers where the relative positions of few atoms differ in the molecule in the three-dimensional space because of the rotation about sigma bonds.
- Configurational isomerism
Configurational isomerism is caused due to remaking and breaking of covalent bonds. They are further divided into:
- Geometrical isomerism
- Optical isomerism
Configuration
In the context of stereochemistry, the term is restricted to the arrangements of atoms of a molecular entity in space that distinguishes stereoisomers, the isomerism between which is not due to confirmation differences.
- Absolute configuration
It is the spatial arrangement of the atoms of a chiral
Molecular entity (or group) and its stereochemical description e.g., R or S.
- Relative configuration
The configuration of any stereogenic (asymmetric) centre with respect to any other stereogenic centre contained within the same molecular entity. Unlike
Absolute configuration, the relative configuration is a reflection-invariant. Relative configuration, distinguishing diastereoisomers, may be denoted by the configurational descriptors R*R*, R*R* (or l) and R*R*, S*S* (or u) which means, respectively, that the two centers have similar or opposite configurations. For molecules that have more than two
Asymmetric centers the prefix rel- may be used in front of the name of one
Enantiomer where R and S have been used. If any centres have known
Absolute configuration then only R*R* and S*S* can be used for the relative configuration.
The symmetry of a molecule describes how its different parts relate to one another geometrically. Symmetry plays an important role in many areas of chemistry, with effects on:
- Physical properties: e.g., dipole moment, chirality
- Spectroscopic properties: geometric equivalence of groups or nuclei, transition intensities
- Bonding interactions: the bonds need to overlap of atomic orbitals of correct symmetry
- Symmetry Operation: a spatial manipulation performed on a molecule that leaves it in a configuration identical to and superimposable upon the original configuration.
Symmetry Element: It is an axis, point, or plane about which a symmetry operation is performed.
Point Group: It is a symbol that identifies all the symmetry elements present in a molecule.
Symmetry Elements and Operations |
Element | Operation | Symbol | ANotes |
None | Identity | E |
|
Proper | Rotate by | Cn |
|
Mirror plane | Reflection | Σ |
|
Inversion | Inversion | I |
|
Improper | Rotate by 360º/n, then reflect ^ to the axis | Sn |
|
Effects on Molecular Properties |
- Chirality:
For a molecule to be chiral, both the functions Inversion (i) and Reflection(σ) must be absent. * Those Molecules that belong to groups Cn (including E) and Dn are chiral. * This is a small simplification. The most accurate requirement for chirality is the lack of any Sn element, as the appearance of these is difficult to figure out, however, there are a series of increasingly precise shortcuts used to Spotting a Chiral Compound.
There are two types of STEREOISOMERS, enantiomers, and diastereomers.
Enantiomers contain chiral centers that are non-superimposable & mirror images. They only come in pairs!
Diastereomers contain chiral centers that are non-superimposable but are NOT mirror images. There can be more than 2 depending on the number of stereocenters.
An easy way to remember enantiomers from diastereomers is to analyze and study the picture given below. In cases where there are 2 chiral centers, 4 stereoisomers are possibly formed. Only the exact opposites (diagonal arrows) are enantiomers and they, therefore, have a mirror image that is not superimposable. The molecules with only one stereocenter that differs (parallel arrows) are diastereomers.
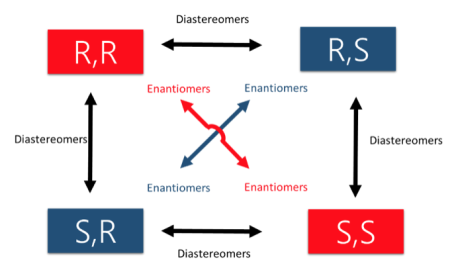
Fig.2: The picture shows two chiral centres, resulting in the formation of 4 stereoisomers. The diagonal arrows are enantiomers, their images are not superimposable though they are mirror images formed, the parallel arrows are diastereomers with only one stereocentre.
A biological example of this property can be viewed in, saccharide (or sugar), and below are the enantiomers and diastereomers of threose molecule.
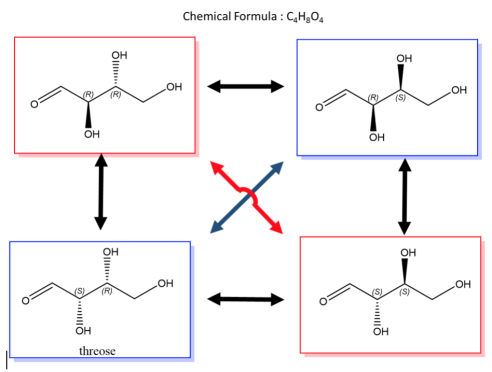
Fig.3: The picture shows a molecule of 5-DHT, an active form of Testosterone and has 7 stereocentres, Using the formula, 128 possible combinations are formed, only one is an enantiomer the rest are stereoisomers.
While enantiomers are found to only come in pairs, many diastereomers can exist without a given molecule. Let’s take, 5-DHT for example, this compound is the metabolically active form of testosterone and has 7 stereocenters, using the 2N rule for determining the number of stereoisomers, we get 128 possible combinations from the given formula. But only one of them is the enantiomer of 5DHT! The rest are diastereomers.
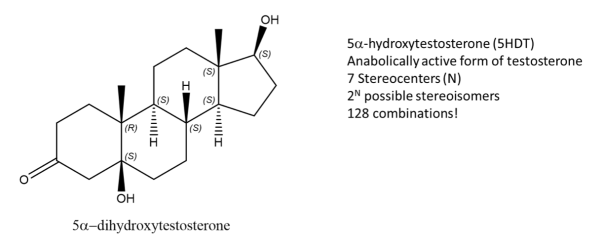
Fig.4: Structure of Testosterone, using the 2n rule, 128 combinations are formed.
The optical activity of a substance is the tendency of a substance to rotate along the plane of polarization of a beam of light that is passed through it. (In plane-polarized light, the vibrations of the electric field are confined to a single plane.) The intensity of optical activity of a substance is expressed in terms of a quantity, called specific rotation, the specific rotation is defined by an equation that is related to the angle through which the plane is rotated, the length of the light path through the sample, and the density of the sample (or its concentration if it is present in a solution). As the specific rotation depends upon the temperature and upon the wavelength of the light, these quantities also must be specified. The rotation is assigned a positive value if it is clockwise with respect to an observer facing the light source, negative if counterclockwise. A substance with a positive specific rotation is described as dextrorotatory and denoted by the prefix d or (+); a substance with a negative specific rotation is laevorotatory, designated by the prefix l or (-).
The optical activity was first discovered in quartz, crystals by a French physicist in 1811, Francois Arago. Another French physicist, Jean-Baptiste Biot, found in 1815 that liquid solutions of tartaric acid or sugar are optically active, as are liquid or vaporous turpentine. However, Louis Pasteur was the first to recognize that optical activity appears from the dissymmetric arrangement of atoms in the crystalline structures or also in individual molecules of certain compounds.
Configuration can be defined as an arrangement of elements or parts in a particular figure, form, or found in any combination. It refers to the spatial arrangement of the atoms of a particular chiral molecular entity (or group) and its stereochemical description.
e.g., R or S, which refer to as Rectus, or Sinister,
Respectively. Absolute configurations for a chiral molecule (in pure form) are most
Often obtained through X-ray crystallography.
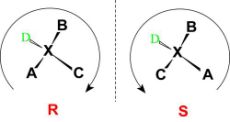
Fig:5: The figure shows the R and S configurations to assign the absolute configuration to a structure.
Conformational Analysis
Conformation Analysis is related to any of the spatial arrangements of the atoms in a molecule these atoms may adopt and freely convert between, especially by rotation about individual single bonds.
It’s a branch of stereochemistry that studies the conformations of molecules and their relations to the chemical and physical properties of substances. The Dutch chemist J.H.Van’t Hoff based on the stereochemical hypothesis he formulates (1874-75) on two principal postulates: (1) the valences of the saturated carbon atom are directed in space toward the vertices of the tetrahedrons, and (2) the atoms or groups of atoms (substituents) in the molecule can freely rotate about single bonds without being broken (unlike double bonds whose rigidly causes the formation of geometric isomers). Subsequently, the tetrahedral model of the carbon atom was confirmed by direct X-X-ray analysis. The supposition regarding the free rotation about single bonds was subjected to review, as it is already established that rotation about single bonds is not entirely “free”; during such rotation, energetically unequal geometric forms-conformations-or rotational isomers are formed, some of which are energetically more favourable than others, will emerge. Most of the atoms present in molecules are present primarily in one or several stable (preferred) conformations.
Different conformation of the same substance that is separated by energy barriers are usually equal to 20.9-62.7 kilojoules per mole (5-15 kilocalories per mole); individual conformations constantly convert into one another. The investigations performed by the British chemist D. Barton on the conformations in the cyclohexane series were of singular importance; Barton also introduced the term “conformational analysis” in (1950).
In the paraffin hydrocarbon series, the necessity of conformational analysis is another evidence in the case of ethane, that have two possible conformations: the eclipsed (or even) and staggered (or odd). These are formed during the rotation of one methyl group with respect to another (Figure 1).
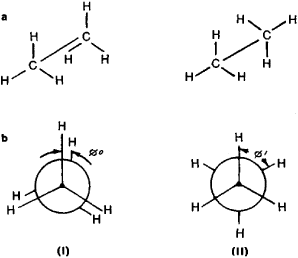
Figure 6. (a) Perspective formulas and (b) Newman’s projection (view of a molecule from “above” along with the C—C bond) formulas, depicting the even or eclipsed (I) and staggered or odd in (II) conformation of Ethane. ø is the angle between the substituents also known as the dihedral angle
Conformational representations have great importance in the chemistry of synthetic and natural macromolecular compounds, as well as in the field of physiologically active substances.
In the organic chemistry and also inorganic chemistry, these are fundamental reactions that occur when a leaving group is replaced by an electron-rich compound (nucleophile), nucleophilic substitution reaction is a class of reactions, when the whole entity of the leaving group and the nucleophile together is called the substrate. a fundamental class of reactions in which a leaving group is replaced by an electron-rich compound (nucleophile). The nucleophile attempts to replace the leaving group as the primary substituent in the reaction itself, as a part of another molecule.
The most general form of the reaction may be given as the following:
Nuc: + R-LG → R-Nuc + LG:
The nucleophile (Nuc) that possess the electron pair (:), forms a new bond by attacking the substrate (R-LG), the substrate while leaving (LG) has an electron pair. The main product formed here is R-Nuc. The nucleophile formed is either electrically neutral or negatively charged, but the substrate is typically neutral or positively charged.
An example of nucleophilic substitution is, under basic conditions the hydrolysis of alkyl bromide-Br, in this case, the attacking nucleophile is the OH- and the group that leaves is Br-.
R-Br + OH− → R-OH + Br−
SN1 is a unimolecular substitution reaction, it involves the separation of a negatively charged functional group of atoms. This results in the formation of a carbocation. The anion or the other negatively charged functional group or atom then gets attached to the carbocation. The reaction follows the first order of the kinetics mechanism. It’s a two-step reaction and the rate of reaction depends on the concentration of the substrate.
SN2 is a bimolecular reaction, the reaction happens in a single transition state, the reaction is a single step and follows the second-order of kinetic mechanism. The detachment of the original negatively charged functional group and the attachment of the new negatively charged functional group or anion happen simultaneously forming an intermediate, the replacement gets completed at the end.
Electrophilic Addition Reaction:
The process in which an electrophile (an electron pair acceptor) replaces the functional group attached to a compound is called an electrophilic substitution, the displaced substitution group is usually a hydrogen atom. Electrophilic substitution occurs in many reactions of arenes (a compound containing Benzene rings), and it is called electrophilic aromatic substitution reactions. The electrophilic aliphatic substitution reaction is another primary type of electrophilic reaction,
It is a three-step process that involves
- Generation of an electrophile
- Formation of carbocation that acts as an intermediate
- Removal of a proton from the medium.
Nucleophilic Addition Reaction:
A nucleophilic addition reaction is a chemical addition reaction in which a nucleophile forms a sigma bond with an electron-deficient species. These reactions are considered very important in organic chemistry since they enable the conversion of carbonyl groups into a variety of functional groups. Generally, nucleophilic addition reactions of carbonyl compounds can be broken down into the following three steps.
- The electrophilic carbonyl carbon forms a sigma bond with the nucleophile.
- The carbon-oxygen pi bond is now broken, forming an alkoxide intermediate
- The subsequent protonation of the alkoxide yields the alcohol derivative.
The carbon-oxygen double bond is directly attacked by strong nucleophiles to give rise to the alkoxide. However, when weak nucleophiles are used, the carbonyl group must be activated with the help of an acid catalyst for the nucleophilic addition reaction to proceed.
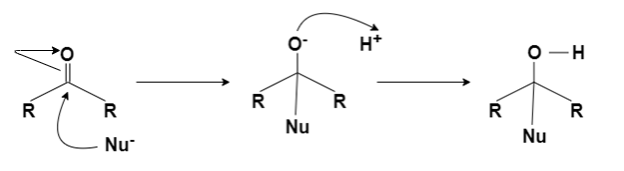
The carbonyl group has a coplanar structure and its carbon is sp2 hybridized. However, the attack of the nucleophile on the C=O group results in the breakage of the pi bond. The carbonyl carbon is now sp3 hybridized and forms a sigma bond with the nucleophile.
The compound Propene belongs to the alkene compound group. Alkyl bromide compound is produced when propene readily reacts with HBr.The reaction that occurs is an example of a nucleophilic addition reaction.
Propane is an Alkene with three carbon atoms and is unsymmetrical, the major product formed when propene reacts with HBr is 2-bromopropane and 1-bromopropane is the minor product formed.
CH3CH=CH2 + HBr → CH3CHBrCH3
CH3CHBrCH3 (2-bromopropane) is the major product formed.
IN the given reaction HBr molecule is added across the double bond of propene. Marconikov rule is followed in this reaction, which helps in finding the locations of the bromine and hydrogen atoms added and carbon atoms in the double bond.
Morkownihoff Rule:
On addition of HX(X= Halide elements) to an asymmetrically substituted alkene, the addition of acidic hydrogen takes place at the less substituted carbon atom of the double bond, while halide X is added to the more alkyl substituted carbon atom. In other words, hydrogen is added to the carbon atom with more hydrogen atoms attached to it, and halide is added to the carbon atom with the least number of hydrogen atoms.
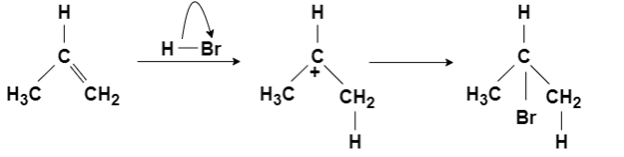
The reaction proceeds for the formation of carbocation by the addition of H to the alkenes, in the first step of the reaction. The most stable carbocation is formed when H is added to carbon having more number of hydrogen atoms already attached – due to factors like induction and hyperconjugation – which gives the major product with Br added to less hydrogen-rich carbon. The less stable carbocation is also present in small quantity forming minor products on the addition of Br to carbon having more hydrogen attached to it.
Grignard reagents are usually alkyl, vinyl, or aryl magnesium halides that are added to any carbonyl group in a ketone or an aldehyde. The reaction is important in the formation of carbon-carbon bonds. The name Grignard is named after – French scientist Francois Auguste Victor Grignard, who was awarded the Nobel Prize in Chemistry in 1912 for this discovery.
A Grignard reagent is a strong nucleophile and can behave like carbonyl compounds with electrophiles.
Grignard Reaction Mechanism
The mechanism involves the attack of this reagent on the electrophilic carbon present in the polar bond of the carbonyl group., the process is carried out through a six-membered ring transition state.

Some of the processes with Grignard may carry out a single electron transfer process, others may involve the formation of a carbon-phosphorus bond, carbon-silicon bond, and carbon-boron bond.
When the reagent combines with a ketone in a rate-controlled step, simultaneous displacement of the ether molecule occurs. In a six-membered Transition state the monomeric Grignard reagent undergoes a rapid second reaction to form alcohol. It has been proposed that the reaction can proceed with RMgX, RMg, or both of these organometallic compounds.
Elimination reaction is the type of reaction that is mainly used to convert saturated compounds to unsaturated compounds that is organic compounds that contain single carbon bonds to the compound which features double or triple carbon bonds. It is a chemical reaction where several atoms either pair or groups are removed from a molecule. The removal usually takes place due to the action of acids and bases or the action of metals. It can also happen through the process of heating at high temperatures.
Mechanism
The elimination reaction consists of three fundamental events they are:
- C-C pi bond is formed.
- Proton removal.
- There is a breakage in the bond of the leaving group.
Depending on the reaction kinetics, elimination reactions can occur mostly by two mechanisms namely E1 or E2 where E is referred to as elimination and the number represent the molecularity.
E1 Reaction
This is also called as a unimolecular elimination reaction, there are usually two steps involved – ionization and deprotonation.
During ionization, there is a formation of carbocation as an intermediate. In deprotonation, a proton is lost by the carbocation.
This happens in the presence of a base which further leads to the formation of a pi-bond in the molecule.
In E1, the reaction rate is also proportional to the concentration of the substance to be transformed.
It exhibits first-order kinetics.
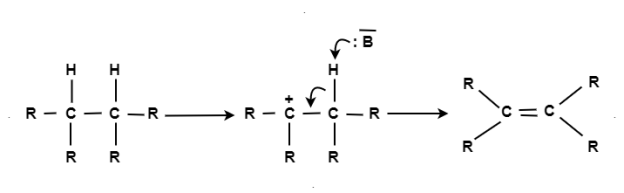
E2 Reaction:
An E2 mechanism which refers to bimolecular elimination is a one-step mechanism.
Here, the carbon-hydrogen and carbon-halogen bonds mostly break off to form a new double bond.
However, in the E2 mechanism, a base is part of the rate-determining step and it has a huge influence on the mechanism.
The reaction rate is mostly proportional to the concentrations of both the eliminating agent and the substrate.
It exhibits second-order kinetics.
The E2 mechanism can generally be represented as below. In the below-mentioned representation, B stands for base and X stands for the halogen.
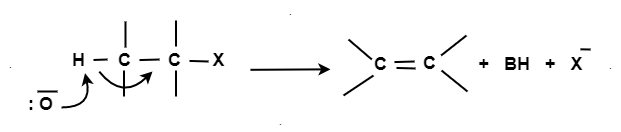
In organic chemistry, Saytzeff rule is an empirical rule to predict the alkene product that is favoured during an elimination reaction. The rule is named after Russian chemist Alexander Zaitsev, who studied a variety of elimination reactions and observed a specific trend in the alkenes, based on this trend, He stated "The alkene formed in the greatest amount is the one that corresponds to the removal of the hydrogen from the alpha-carbon having the fewest hydrogen substituents’
’For example, when 2-iodobutane is treated with alcoholic potassium hydroxide, the major product formed is 2-butene and minor product is 1-butene
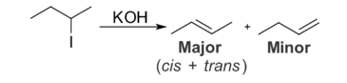
In general, the Saytzeff rule signifies that in an elimination reaction, the product that is more substituted will be the more stable and most favoured one. The rule makes no generalizations of the newly formed alkene with respect to stereochemistry, but only specifies the Regio chemistry of the reaction. While the rule has many exceptions, it is effective in predicting the favoured product for many elimination reactions.
4.16 Oxidation reactions: Oxidation of alcohols using KMnO4 and chromic acid
The important step in the synthesis is the oxidation of an alcohol to form an aldehyde or ketone is very important in synthesis. In this process, the hydroxy hydrogen of the alcohol is replaced by a leaving group (X in the figure below).
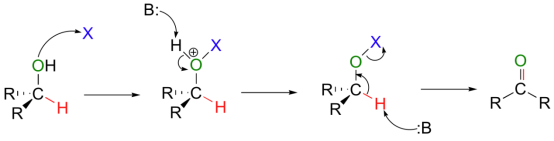
Then, a base can abstract the proton bound to the alcohol carbon, which results in the elimination of the X leaving group and the formation of a new carbon-oxygen double bond. there is no hydrogen to abstract in the final step.
Oxidation using chromic acid
Chromic acid is also known as Jonas reagent and is obtained by adding chromium trioxide (CrO3) to aqueous sulfuric acid, chromic acid is used as the oxidizing agent for oxidizing secondary alcohols to ketones. This oxidation method also uses acetone in the reaction, which helps in preventing excess oxidation of the organic product.
A mechanism for the chromic acid oxidation of a ketone is shown below.
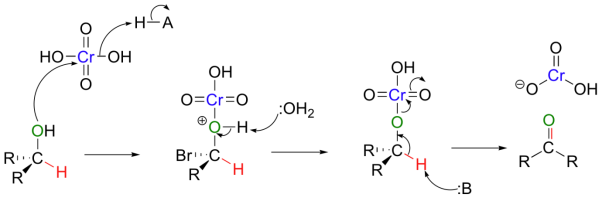
The chromium reagent has lost two bonds to oxygen in this reaction and thus has been reduced.
Ketones are not oxidized by chromic acid, so the reaction stops at the ketone stage. In contrast, primary alcohols are oxidized by chromic acid first to aldehydes, then straight on to carboxylic acids.
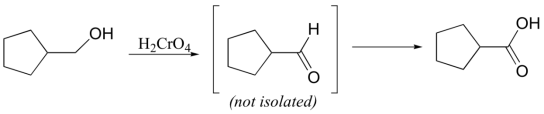
It is actually the hydrate form of the aldehyde that is oxidized:
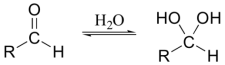
One of the hydroxyl groups of the hydrate attacks chromic acid, and the reaction proceeds essentially as shown for the oxidation of a secondary alcohol.
Under some conditions, chromic acid will even oxidize carbon in the benzylic position to a carboxylic acid (notice that a carbon-carbon bond is broken in this transformation), either chromic acid or KMnO4 is suitable, and they give the same carboxylic acid product.
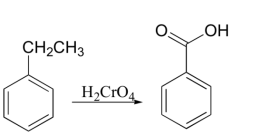
Alcohols are usually obtained by the reduction of ketones, esters, aldehydes, and other carbonyl compounds, numerous reagents reduce carbonyls to alcohols they include LiAlH4, BaBH4, DIBAL. The reagents used have both advantages and disadvantages, some are selective and others are powerful in nature.
Lithium Aluminium Hydride LiAlH4 is the stronger ‘common’ carbonyl reducing agent. This reagent is also known to reduce carboxylic acids and carboxyl derivatives, in addition to reducing aldehydes and ketones like NaBH4.
In reductions that involve metal hydrides, the reductions the resulting alkoxide salts formed are insoluble and should be hydrolyzed (with care) before the alcohol product can be isolated. However, in sodium borohydride reduction, this hydrolysis is automatically achieved with a methanol solvent system. In the lithium aluminium hydride reduction, in the second step of the reaction water is usually added. The lithium, sodium, boron, and aluminium end up as soluble inorganic salts at the end of either reaction. Note that LiAlH4 and NaBH4 are both capable of reducing aldehydes and ketones to the corresponding alcohol.
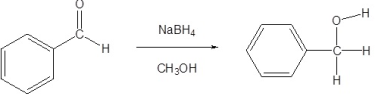
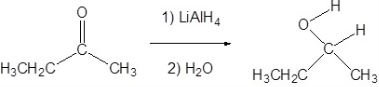
The hydroboration of olefins, dienes, and acetylenes involves the addition of a boron-hydrogen bond to the carbon-carbon multiple bonds. This reaction provides a new, convenient route to the corresponding organoboranes and makes them readily available as intermediates in organic synthesis. One of the important reactions that the organoboranes undergo is the rapid and essentially quantitative oxidation with alkaline hydrogen peroxide. The hydroboration of olefins involves a cis addition of the boron hydrogen bond, the boron atom becoming attached to the less substituted of the two olefinic carbon atoms of the double bond.

Hydroboration of a terminal alkene to a trialkyl borane, showing an idealized image of the cyclic transition state.
Aspirin:
The chemical name for the Aspirin is Acetylsalicylic acid. It is used as a pain killer and fever reducer. Salicylic acid derives from the willow family of plants which were widely used for treating headaches. For the preparation of aspirin, the salicylic acid is reacted with the excess acetic anhydride. Phosphoric acid is used for boosting the procedure of reaction. The excess acetic acid will be quenched with the addition of water. The aspirin product is not very soluble in water so the aspirin product will precipitate when water is added. The synthesis reaction of aspirin is shown below:
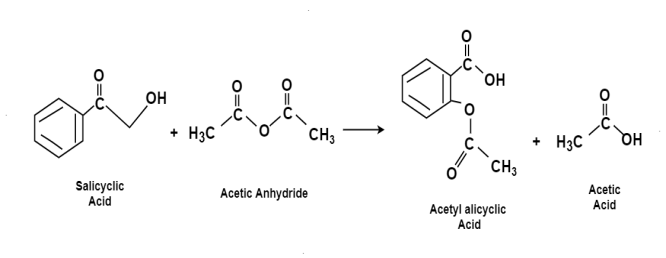
Since acetic acid is very soluble in water, it is easily separated from the aspirin product. The aspirin isolated in this step is the “crude product”. A “purified product” can be obtained through recrystallization of the crude product in hot ethanol. In this experiment, the crude product will be the desired product. The percent yield of the crude product will be determined for this reaction. The purity of the product will also be analyzed. The product will be analyzed by three different methods: melting point, titration, and spectroscopic assay. C and the melting point range of the salicylic acid. The melting point range of pure aspirin is 138-140 C. If impurities are present in your crude sample, the melting point rangestarting material is 158-161 for your product will be lower than the range of pure aspirin. Also, your melting point range may be greater than 2 degrees.
Paracetamol:
From Phenol:
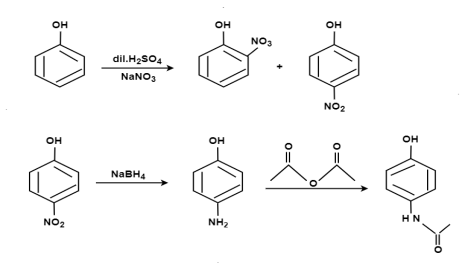
From Para nitro Phenol:
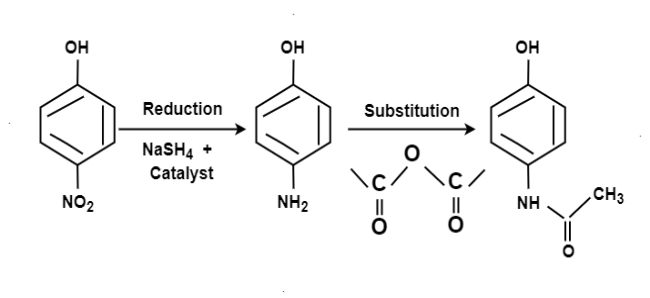
References:
- Introduction to Stereochemistry: Kurt Mislow.
- Organic Chemistry: Ajay Yadav.
- General, Organic and Biochemistry: James Armstrong.