Unit - 5
Three Phase Induction machine
- An electrical motor, which operates on a.c. Supply is called AC motors.
- As AC supply is commonly used, AC motors are very popular.
- AC motor are classified as 1 & 3
- IM synchronous motors and some special purpose motors.
- Out of these 3 IM are widely used for various industrial applications.
- The advantage of B - IM, over other types are self-starting properly i.e. no need of starting device, higher P.F., good speed regulations and robust construction.
Construction:
I.M. Consists of two main parts
1. Stator
Stationary 3 winding Part Called ‘stator’
2. Rotor
Rotating part & is connected to mech. Load through shaft called ‘Rotor’.
1) Stator
The stator has laminated type of construction made up of stamping which are 0.4 to 0.5 mm thick.
a) The stampings are slotted on its periphery to carry stator windings the stampings are insulated from each other.
b) The no. Of stampings is stamped together to build stator core.
c) The built-up core is then fitted in casted or Fabricated steel frame. The material of stampings is silicon steel because it minimizes hysteresis loss.
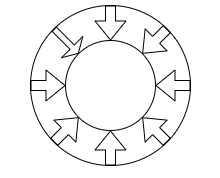
d) The stops on periphery of stator core carries a 3 winding, connected either in star or delta, this 3 winding is called stator winding.
e) The radial ducts are provided for cooling purpose.
2) Rotor
- The rotor is placed inside stator.
- The air gap between stator and rotor is 0.4 mm to 4 mm.
There are two types rotor Construction.
a) Squirrel cage rotor
b) Slip ring or phase wound
a) Squirrel cage rotor:
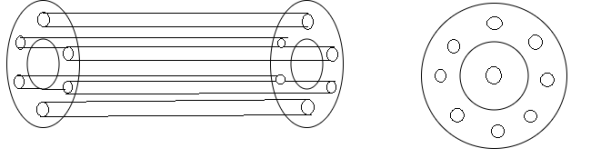
a) The rotor core is cylindrical and slotted on its periphery.
b) The rotor consists of uninsulated cu or Al bars called ‘rotor conductors. The bars are paced in the armature slots.
c) These bars are permanently shorted at each end with the help of conducting ring called ‘end ring’. The bars are brazed to end rings to provide good mechanical strength.
d) The entire structure looks like a cage, forming a closed ckt, so rotor is called squirrel cage rotor.’
e) As the bars are permanently shorted to each other though end ring, the entire rotor resistance is very small. Hence rotor is called ‘short circuited rotor’.
f) As rotor itself is short drauited, no external resistance can have effect on rotor resistance so external resistance is inserted.
g) In this type of rotor, slots are not arranged parallel to shaft axis but they are skewed.
Advantages of Skewing:
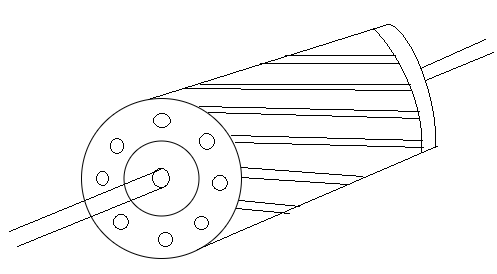
a) Magnetic hum (noise) gets reduced
b) Makes motor operation smooth.
c) Stator and rotor teeth may get magnetically locked, due to skewing it is reduced almost.
d) Increases the effective transformation ratio between stator and rotor.
b) Slip Ring Rotor or Phase wound Rotor:
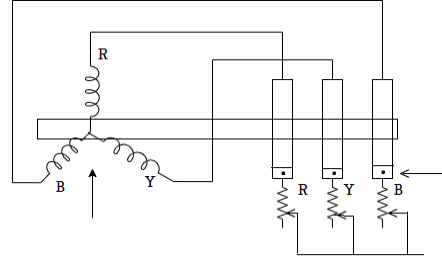
a) In this type, rotor winding is exactly similar to stator the rotor carries 3 Y or connected, distributed winding wound for some number of poles as that of stator.
b) The rotor construction is terminated and slotted, the slots contains the rotor winding.
c) The 3 ends of 3 winding available after connected the winding in Y or are permanently connected slip rings.
d) With the help of slip rings, external resistance can be added in series with each phase of rotor winding.
e) In running condition, the slip rings are shorted
f) The possibility of addition of external resistance in series with rotor, is the main feature of this type rotor.
Difference Between
Slip Ring/ Wound | Squirrel Cage |
1) Rotor and stator have similar 3 winding | 1) Rotor consists of bars with end rings |
2) Construction is complicated | 2) Construction is very simple |
3) External resistance can add | 3) External resistance can’t add |
4) Rotors are very costly | 4) Rotors are cheap |
5) Slip ring and brushes are present | 5) Slip ring and brushes are absent |
6) High starting torque can be detailed | 6) Moderate starting torque. |
Working Principles
A. IM works on principle of electromagnetic induction.
B. When 3 supply is given to 3 stator winding, a rotating magnetic field of constant magnitude is produced, the speed of this rotating magnetic field is synchronous speed (NS) in rpm.
= Speed of rotating magnetic field.
C. This rotating magnetic field produces on effect of rotating poles around a rotor.
D. Let direction of rotation of this rotating magnetic field is clockwise. (Fig a)
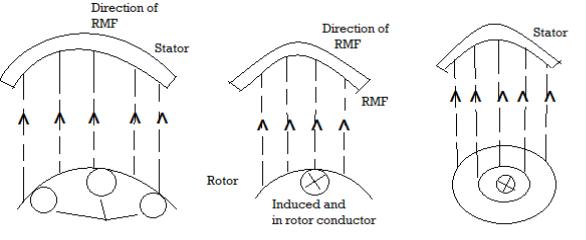
Now at this instant rotor is stationery and stator flux RMF is rotating. So, it’s obvious that there exists a relative motion between RMF and motor conductors.
E. Whenever conductor cuts the flux, emf gets induced in it.
F. As rotor forms closed circuit, induced emf circulates current though rotor called rotor current fig.(b).
G. Any current carrying conductor produces its own flux, called rotor flux fig. (c).
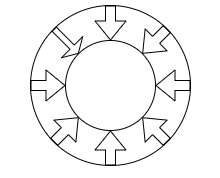
H. Both fluxes interact each other as shown in fig (d)
I. On left of rotor conductor, two fluxes are in same direction hence add each other. While on ri9ght side of rotor, fluxes cancel each other to produce low flux area.
J. High flux density area exerts a push on rotor conductor towards low flux density area, so rotor conductor experiences forces from left to right fig. (d)
K. As all rotor conductors experience a force, the overall rotor experiences a torque and start rotation so interaction of fluxes is very essential for a motion action.
L. The cause of rotor current is the induced E.M.F which is induced because of relative motion present between rotating magnetic field and rotor conductors.
M. To oppose relative motion i.e. to reduce relative speed, rotor experiences a torque in same direction as that of RMF and tries to catch up the speed of rotating magnetic field.
N. NS = Speed of rotating magnetic field.
N = Speed of rotor i.e. motor speed in rpm (NS – N) = Relative speed between two, rotating magnetic field and rotor.
O. Thus, rotor always rotates in same direction as that of RMF. (N < NS).
Slip of Induction Motor

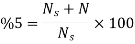
N = NS()
Can NS = N
Also, Never NS> N
Effect of slip on Rotor Parameters
The slip affects the rotor parameters when an I.M runs with speed N rpm.
The various parameter which gets affected by the slip are-
- F
- Induced emf
- Current
- p.f
- Impedance
1) Effect on Rotor Frequency
At start speed of I.M. Is zero (N = 0) & slip s = l.
Then the frequency of rotor induced emf is same as the stator supply frequency.
If
Fr = Frequency of rotor induced emf
f = Stator supply frequency
Fr = F at start when S = l.
But when motor rotates at speed N, there exists a slip S & then in running condition, frequency of rotor induced emf is slip times the stator supply frequency.
Fr = Sf … in running condition
As slip is small, Fr is very small in
2) Effect on magnetic of rotor induced emf
Let
E2 = Rotor induced emf/ Ph in stand still
While
E2r = Rotor induced emf/ Ph in running
The induced emf depends on relative motion between rotor &RmF.
It is max. At start hence E2 is max at start.
In running condition, relative speed becomes Ns – N hence induced emf reduce, by slip times. The max. Emf E2 at stand still.
E2r = SE2
3) Effect on Impedance
The rotor imp. Has two comp. Resistance and inductive reactance.
The XL depends on the frequency.
Let,
R2 = Rotor resistance/ ph. On standstill condition.
X2 = Reactance
Z2 = R2 + jx2 = Rotor imp. On stand.(N = 0)
In running condition, rotor frequency. (Fr = SF)
Hence,



Hence rotor imp. In running condition (Z2r) is
Z2r = R2 + jx2r = R2 + js x 2
4) Effect on Rotor P.F.
The P.F. Is the ratio of R toZ
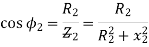
While in running condition, changes to
. Hence P.F. In running condition is given by
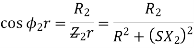
5) Effect on rotor current
The ratio of rotor induced emf to rotor imp. Is the rotor current.
Hence rotor current on stand still is
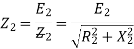
While in running condition, E2 changes to SE2 and changes to Z2r hence rotor current becomes.
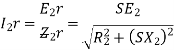
The fundamental principle of induction machine is the creation of rotating and sinusoidally distributed magnetic field in the air gap. Three phase balanced power supply is fed to the three-phase stator winding creates a synchronously rotating magnetic field. Due to relative speed between rotating flux and stationary conductors, an emf is induced. The frequency to induced emf is same as supply frequency.
As shown in below fig.a , the stator field is assumed to clockwise. The relative motion of rotor w.r.t stator is anticlockwise. From Right-hand rule, the direction of induced emf in rotor is outwards. By the effect of combined field as shown in fig b the rotor experiences a force tending to rotate it in clockwise direction. Hence rotor rotates in the same direction as the stator field.
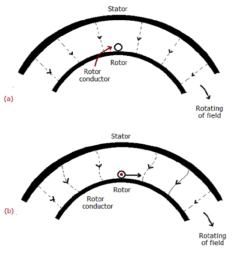
Fig. Rotating Magnetic Field
The speed of this rotating field is called synchronous speed. If the rotor is initially stationary, its conductors will be subjected to a changing magnetic field, inducing current in the short-circuited rotor at the same frequency. The interaction of air gap flux and rotor mmf produces torque. At synchronous speed rotor cannot have any torque.
Consider a 2 pole synchronous motor. When a three phase AC voltage is applied to the stator winding, a rotating magnetic field is produced in the air gap. The stator field rotates at synchronous speed.
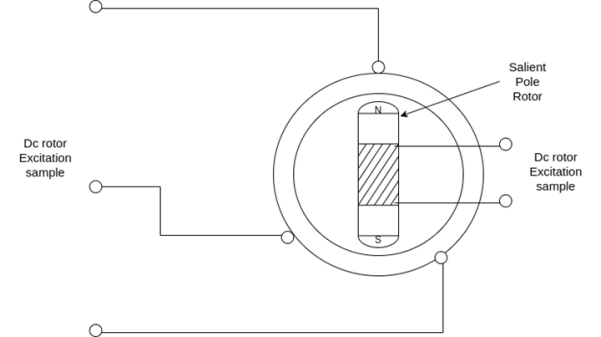
Fig. Synchronous Motor
The field current of the motor produces a steady state magnetic field. Therefore there are two magnetic fields present in the machine. The rotor will tend to align if placed near each other to the stator field.
Since the stator magnetic field is rotating the rotor magnetic field and the rotor will tend to rotate with the rotating field of the stator.
In order to develop a continuous torque, the two fields must be stationary with respect to each other. This is possible when the rotor also rotates at synchronous speed.
The basic principle of synchronous motor operation is that the rotor chases stator magnetic field. In other words the stator rotating magnetic field tends to drag the rotor along, as if north pole on the stator locks in with the South Pole of the rotor.
When a pair of rotating stator poles sweeps across the stationary rotor poles at synchronous speed, stator poles will tend to rotate the rotor in one direction and then in the other direction.
However, because of the rotor inertia the stator fields slide by so fast that the rotor cannot follow it. Consequently, the rotor does not move and we say that the starting torque is zero. In other words, a synchronous motor is not self-starting.
Key takeaway
The field current of the motor produces a steady state magnetic field. Therefore, there are two magnetic fields present in the machine. The rotor will tend to align if placed near each other to the stator field.
Since the stator magnetic field is rotating the rotor magnetic field and the rotor will tend to rotate with the rotating field of the stator.
1. As we know the principle of induction motor is very similar to the transformer with some differences.
2. An induction motor at standstill condition is similar to a transformer at no-load condition. Therefore, the method of drawing the induction motor phasor diagram is also the same as that of a transformer phasor diagram.
3. In this we will discuss the phasor diagram of induction motor at standstill condition and full load slip.
Induction Motor Phasor Diagram at Standstill Condition:
Before going into the phasor diagram, there are some important points to be taken care of:
Per phase value of induced emf E1 in the stator winding is given below
E1 = √2πf1kw1N1Ø
Where f1 = supply frequency
N1 = Number of series turns per phase
Ø = resultant air-gap flux per pole
Kw1 = Stator winding factor
Per phase value of induced emf E2 in rotor winding is given as
E2 = √2πf2kw2N2Ø
Where f2 = frequency of induced emf in rotor = sf1
N2 = Number of series turns per phase
Ø = resultant air-gap flux per pole
Kw2 = Rotor winding factor
1. Total air gap mmf Fr of induction motor is the sum of stator mmf (F1) and rotor mmf (F2).
2. Magnetizing current Im took by the stator winding from the supply always remains in phase with the resultant flux Ø. The induced emf always lags behind the resultant flux Ø by 90°.
3. Now we are at a stage to draw the induction motor phasor diagram. Let us take the resultant air-gap flux Ø as the reference. This flux Ø will be in phase with the resultant mmf Fr. Also, the induced emf E1 and E2 in stator and rotor winding will lag behind the Ø by 90°. This is shown in the below phasor diagram of the induction motor.
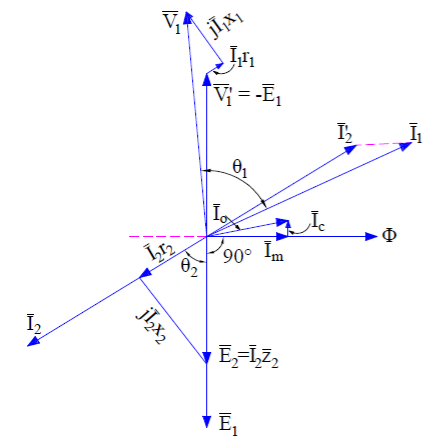
Fig. Phasor diagram of induction motor
4. Since the rotor mmf counteracts the stator produced mmf as per Lenz's law, therefore stator takes extra current from supply to counterbalance the effect of rotor current. Therefore under normal condition,
Stator mmf = Rotor mmf
N1’I2’ = N2’I2
Where N1’ and N2’ are the effective stator and rotor turns per phase.
5. This component of stator current is called the load component. In addition to the load component, the stator also takes magnetizing current Im to build magnetic flux in the air gap.
6. Thus the total stator current I1 = I2’ + Im. This is shown in the above phasor diagram. I2’ is shown the opposite to the rotor current I2 for the reason discussed above.
7. At standstill condition, E2 = I2 (r2 + jx2). The core loss component of stator current Ic is in phase with V1’ or –E1. At standstill condition, the friction and windage loss is zero, therefore the stator no-load current is given as
I0 = Im + Ic
Since stator applied voltage V1 must balance the stator back emf V1’ or -E1, stator impedance drop I1(r1 + jx1), therefore we can write
V1 = V1’ + I1(r1 + jx1) ……(1)
A similar equation exists for rotor circuit and can be written as
E2 = I2 (r2 + jx2) ……..(2)
8. The above equations are applied for drawing the induction motor phasor diagram as shown in the above figure. It can be easily seen from the above phasor diagram that, the power factor (cosɵ) of the induction motor at starting is very poor as ɵ is large.
Induction Motor Phasor Diagram at Full Load Slip:
1. At full load, the slip s of induction is low. The stator voltage equation (1) does not change when the motor is loaded. But the rotor voltage equation changes with slip. The rotor induced voltage at any slip s becomes sE2 and the rotor circuit reactance becomes sx2. Therefore,
SE2 = I2 (r2 + jsx2)
2. The above rotor equation when implemented, the induction motor phasor diagram will become different from the phasor at standstill condition. The induction motor phasor at full load slip s is shown below.
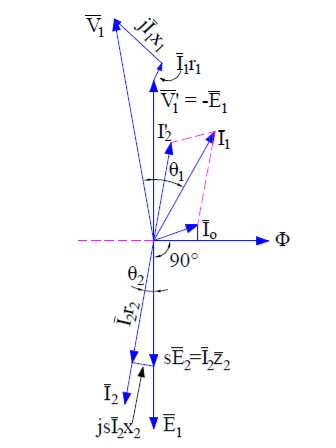
Fig. Phasor diagram at full load
3. Since at full load condition, some friction and windage loss will exist. This means that the stator will have to draw some extra current from the supply main to provide this additional loss.
4. Therefore the total no-load current I0 taken by stator is the sum current Ifc and Im. It can be seen from the above phasor diagram that the power factor of the induction motor improves.
5. Generally at full load the power factor ranges between 0.8 to 0.9.
Key takeaways:
1. The method of drawing the induction motor phasor diagram is also the same as that of a transformer phasor diagram.
2. Magnetizing current Im took by the stator winding from the supply always remains in phase with the resultant flux Ø. The induced emf always lags behind the resultant flux Ø by 90°.
1. The equivalent circuit of any machine shows the various parameter of the machine such as its Ohmic losses and also other losses.
2. The losses are modeled just by inductor and resistor.
3. The copper losses have occurred in the windings so the winding resistance is taken into account. Also, the winding has inductance for which there is a voltage drop due to inductive reactance, and also a term called power factor comes into the picture. There are two types of equivalent circuits in the case of a three-phase induction motor-
Exact Equivalent Circuit
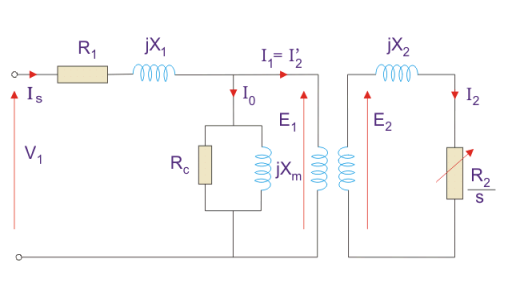
Fig. Equivalent Circuit
Here, R1 is the winding resistance of the stator.
X1 is the inductance of the stator winding.
Rc is the core loss component.
XM is the magnetizing reactance of the winding.
R2/s is the power of the rotor, which includes output mechanical power and copper loss of the rotor.
If we draw the circuit with referred to the stator then the circuit will look like-
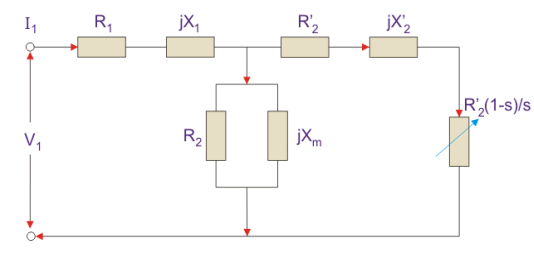
Fig. Equivalent circuit
Here all the other parameters are the same except-
R2’ is the rotor winding resistance referred to as stator winding.
X2’ is the rotor winding inductance referred to as stator winding.
R2 (1 – s) / s is the resistance that shows the power which is converted to mechanical power output or useful power. The power dissipated in that resistor is the useful power output or shaft power.
4. The approximate equivalent circuit is drawn just to simplify our calculation by deleting one node. The shunt branch is shifted towards the primary side.
5. This has been done as the voltage drop between the stator resistance and inductance is less and there is not much difference between the supply voltage and the induced voltage.
However, this is not appropriate due to the following reasons-
1. The magnetic circuit of the induction motor has an air gap so the exciting current is larger compared to the transformer so an exact equivalent circuit should be used.
2. The rotor and stator inductance is larger in the induction motor.
3. In an induction motor, we use distributed windings.
4. This model can be used if the approximate analysis has to be done for large motors. For smaller motors, we cannot use this.
Key takeaways:
1. The winding has inductance for which there is a voltage drop due to inductive reactance and also a term called power factor comes into the picture.
2. This has been done as the voltage drop between the stator resistance and inductance is less and there is not much difference between the supply voltage and the induced voltage.
3. The magnetic circuit of the induction motor has an air gap so the exciting current is larger compared to the transformer so an exact equivalent circuit should be used.
Torque Equation
The torque developed at the moment when motor starts is called as starting torque. As we already know the motor torque Ta α φ Ia. An induction motor develops gross torque Tg due to gross rotor output Pm. Then in terms of rotor input
Tg=
In terms of rotor output the gross torque is Tg=
Due to rotor friction and windage losses the shaft torque Tsh is less than Pm
Tsh= (N, Ns are in rpm)
Tg N-m
Tsh=
N-m
For induction motor the torque is also proportional to the product of flux per stator pole and the rotor current, in addition to the power factor.
Starting Torque
T α φ I2 cos φ2
Or T=k φ I2 cos φ2
I2=rotor current at standstill
φ2=angle between rotor emf and rotor current
E2=rotor emf at standstill.
Also, E2 α φ
So, T α E2I2 cos φ2
T=k1 E2I2 cos φ2
The starting torque Tst= k1 E2I2 cos φ2
From above section we know, =
, cosφ2=
=
Tst= k1 E2 . =
For supply voltage constant , flux and E2 both are constant
Tst=
k1=
Therefore, Tst=
Torque-Slip Relation:
The torque slip characteristics is shown below. For low value of slip the curve is approximately a straight line. As slip increases the torque also increases and becomes maximum when s= R2/X2. This torque is called pull-out or breakdown torque Tb. From above section we already know
T=
T α
When slip increases more with motor load R2 becomes negligible. So, for large value of slip
T α α
Key takeaway
Tg=
Tg N-m
Tst=
T=
Condition for maximum Torque
Torque of rotor in running condition is given as
T== k1
Condition for maximum torque will be obtained by differentiating the above equation, we will get =0
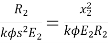
=s2
s=R2/X2
When rotor resistance per phase is equal to rotor reactance per phase under running condition, we have maximum torque. The above torque equation can be written as
Tmax =k1
k1=
Hence, maximum torque is given as
Tmax = N-m
Examples
Que) A 3-phase induction motor star connected rotor has an induced emf of 70volts between slip rings at standstill on open circuit. The rotor has a resistance and reactance per phase of 1ohm and 5ohm respectively. Calculate current/phase and power factor when slip rings are short circuited?
Sol: Standstill emf/rotor phase=70/
Rotor impedance/phase= ohm
Rotor current/phase=40.4/5.09=7.92A
Power factor cosφ=0.99
Que) A 3-phase induction motor star connected rotor has an induced emf of 70volts between slip rings at standstill on open circuit. The rotor has a resistance and reactance per phase of 1ohm and 5ohm respectively. Calculate current/phase and power factor when slip rings are connected to star connected rheostat of 2ohm?
Sol: Rotor resistance/phase=2+1=3 ohm
Rotor impedance/phase==5.83 ohm
Rotor current/phase=(70/)/5.83=6.93A
Cosφ=3/5.83=0.514
Que) A 6-pole, 3-phase induction motor operates from a supply whose frequency is 50Hz. Calculate i)speed at which the magnetic field of stator is rotating. Ii)speed of rotor when slip is 4%. Iii)frequency of rotor current when slip is 3%?
Sol: i)Stator revolves at synchronous speed. So, Ns=120f/P=120 x 50/6=1000rpm
Ii)rotor speed N=(1-s)Ns=(1-0.04) x 1000=960rpm
Iii)frequency of rotor current f’=sf=0.03 x 50=90rpm
Power Equation
For simplicity armature resistance is neglected. Synchronous reactance
and terminal voltage V are assumed constants. The power per phase is given by
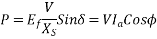
Since V and are constants, therefore for constant power output
and
should remain constant. That is


Also,
When the field current increases the magnitude of increases, but the component of
normal to V, that is,
must remain constant.
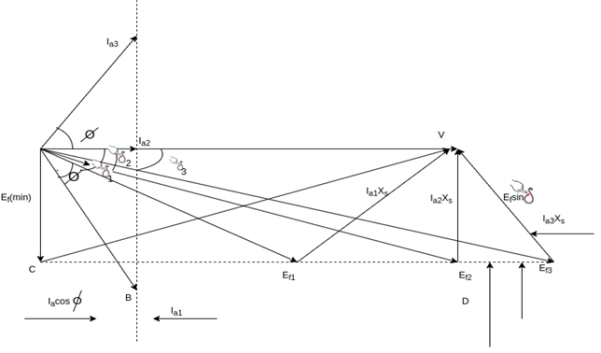
Fig. Effect of varying field current
It is seen that as varies,
and therefore the armature current
also very subject to the condition that
remains constant.
When is varied slowly enough to avoid hunting,
where is in magnitude and the tip of
phases moves along the constant power locus CD so that
remains constant.
Since
And
The above equation shows that the restricted variation of (that is
equal to a constant), the armature current
also varies with the constraint that
remains constant. The tip of the
phasor moves along the constant power locus AB so that
remains constant. Also the phasor
must always remain perpendicular
drop as the tip of the current phasor
moves along its locus.
These three constraints


And is perpendicular to
enable us to draw the phasor diagram of a synchronous motor for varying field currents.
The figure shows the phasor diagram for lagging PF, unity PF, and leading PF.
It is seen in phasor diagram that as the value of increases, the magnitude of the armature current first decreases and increases again. The armature current is minimum at unity PF and more at leading or lagging power factors.
When is small the armature current is lagging and the motor is an inductive load. It acts like an indicator resistor combination, consuming reactive power Q.
As the field current is increased the armature current becomes in phase with the terminal voltage V and the motor becomes a purely resistive load.
As the field current is increased further the armature current becomes leading and the motor becomes a capacitive load. It acts as a capacitor resistor combination consuming negative reactive power -Q or alternately supplying reactive power +Q to the system.
So when



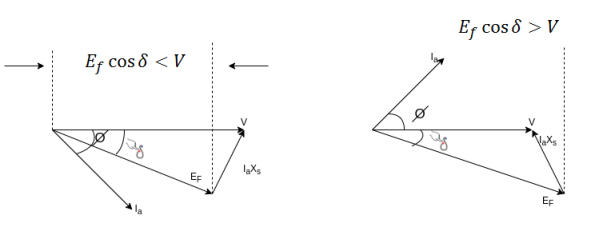
Fig. Phasor Diagram
Losses and Efficiency
The losses are same as those in a transformer
Copper Losses
These are present in windings of the machine. The actual resistance depends on the operating frequency and flux conditions. If there is any difference in these values they are called as stray losses. Brush losses for machines with slip rings are normally neglected and accounted for under the stray loss category.
Mechanical losses
They occur due to the friction in bearings and the energy dissipated in turning the rotor through air inside the machine. Rotational losses are present if there are core losses.
Stray Loss
When we get difference between the actual losses and their calculated values. They occur in brush contact loss.
No-load Core Loss
At no-load hysteresis and eddy current losses are measured. They are also called mechanical losses when combined.
Its efficiency is given by
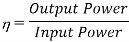
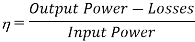
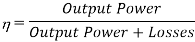
Power Flow
The power flow diagram is shown below for synchronous motor
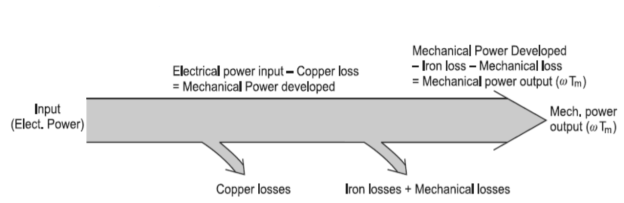
Torque Slip Characteristics of Three Phase Induction Motor
1. The torque slip curve for an induction motor gives us information about the variation of torque with the slip.
2. The slip is defined as the ratio of the difference of synchronous speed and actual rotor speed to the synchronous speed of the machine.
3. The variation of slip can be obtained with the variation of speed that is when speed varies the slip will also vary and the torque corresponding to that speed will also vary.
The curve can be described in three modes of operation-
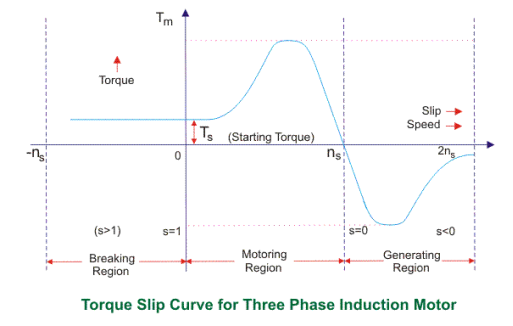
Fig. Torque slip characteristics
The torque-slip characteristic curve can be divided roughly into three regions:
1. Low slip region
2. Medium slip region
3. High slip region
Motoring Mode
1. In this mode of operation, supply is given to the stator sides and the motor always rotates below the synchronous speed. The induction motor torque varies from zero to full load torque as the slip varies. The slip varies from zero to one. It is zero at no load and one at standstill. From the curve, it is seen that the torque is directly proportional to the slip.
2. That is, more is the slip, more will be the torque produced, and vice-versa. The linear relationship simplifies the calculation of motor parameters to great extent.
Generating Mode
1. In this mode of operation induction motor runs above the synchronous speed and it should be driven by a prime mover.
2. The stator winding is connected to a three-phase supply in which it supplies electrical energy. Actually, in this case, the torque and slip both are negative so the motor receives mechanical energy and delivers electrical energy.
3. Induction motor is not much used as a generator because it requires reactive power for its operation.
4. That is, reactive power should be supplied from outside and if it runs below the synchronous speed by any means, it consumes electrical energy rather than giving it at the output. So, as far as possible, induction generators are generally avoided.
Braking Mode
1. In the Braking mode, the two leads or the polarity of the supply voltage is changed so that the motor starts to rotate in the reverse direction and as a result, the motor stops.
2. This method of braking is known as plugging. This method is used when it is required to stop the motor within a very short period.
3. The kinetic energy stored in the revolving load is dissipated as heat. Also, the motor is still receiving power from the stator which is also dissipated as heat.
4. So as a result of which motor develops enormous heat energy. For this stator is disconnected from the supply before the motor enters the braking mode.
5. If the load which the motor drives accelerates the motor in the same direction as the motor is rotating, the speed of the motor may increase more than synchronous speed. In this case, it acts as an induction generator that supplies electrical energy to the mains which tend to slow down the motor to its synchronous speed, in this case, the motor stops. This type of breaking principle is called dynamic or regenerative breaking.
Key takeaways:
1. The variation of slip can be obtained with the variation of speed that is when speed varies the slip will also vary and the torque corresponding to that speed will also vary.
2. The induction motor torque varies from zero to full load torque as the slip varies. The slip varies from zero to one.
3. Induction motor is not much used as a generator because it requires reactive power for its operation.
4.. If load which the motor drives accelerates the motor in the same direction as the motor is rotating, the speed of the motor may increase more than synchronous speed
1. The impedance of magnetizing path of the induction motor is large enough to obstruct the flow of current. Therefore, a small current is applied to the machine due to which there is a fall in the stator-impedance value, and rated voltage is applied across the magnetizing branch.
2. But the drop in stator-impedance value and power dissipated due to stator resistance is very small in comparison to the applied voltage. Therefore, their values are neglected and it is assumed that total power drawn is converted into core loss.
3. The air gap in a magnetizing branch in an induction motor slowly increases the exciting current and the no-load stator I2R loss can be recognized.
4. One should keep in mind that the current should not exceed its rated value otherwise rotor accelerates beyond its limit.
5. The test is performed at poly-phase voltages and rated frequency applied to the stator terminals. When the motor runs for some time and bearings get lubricated fully, at that time readings of applied voltage, input current, and input power are taken.
6. To calculate the rotational loss, subtract the stator I2R losses from the input power.
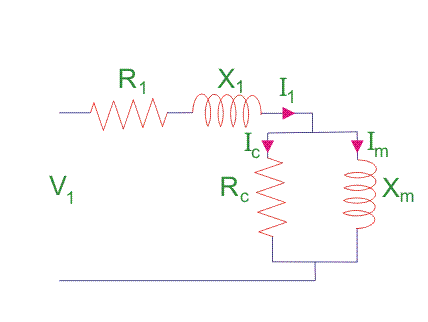
Fig. No-load test
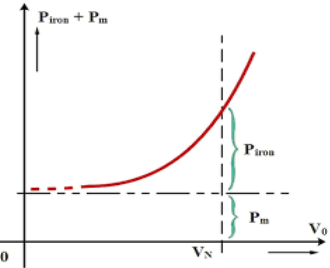
Fig. Graph for no-load test
Calculation of No-Load Test of Induction Motor
Let the total input power supplied to the induction motor be W0 watts.
…….(1)
Where,
V1 = line voltage
I0 = No load input current
Rotational loss
Where,
S1 = stator winding loss
Nph = Number phase
The various losses like windage loss, core loss, and rotational loss are fixed losses that can be calculated by
Stator winding loss = 3Io2R1
Where,
I0 = No load input current
R1 = Resistance of the motor
Key takeaways:
1. The impedance of magnetizing path of induction motor is large enough to obstruct the flow of current
2. One should keep in mind that the current should not exceed its rated value otherwise rotor accelerates beyond its limit.
1. The induction motors are widely used in industries and consume maximum power. To improve its performance characteristics certain tests have been designed like no-load test and block rotor test, etc.
2. A blocked rotor test is normally performed on an induction motor to find out the leakage impedance.
3. Apart from it, other parameters such as torque, motor, short-circuit current at normal voltage, and many more could be found from this test. The blocked rotor test is analogous to the short circuit test of the transformer.
4. Here shaft of the motor is clamped i.e. blocked so it cannot move and rotor winding is short-circuited.
5.In slip ring motor rotor winding is short-circuited through slip rings and in cage motors, rotor bars are permanently short-circuited.
6. The testing of the induction motor is a little bit complex as the resultant value of leakage impedance may get affected by rotor position, rotor frequency, and magnetic dispersion of the leakage flux path.
7.These effects could be minimized by conducting a blocking rotor current test on squirrel-cage rotors.
Process of Testing of Blocked Rotor Test of Induction Motor
1. In the blocked rotor test, it should be kept in mind that the applied voltage on the stator terminals should be low otherwise normal voltage could damage the winding of the stator.
2. In the block rotor test, the low voltage is applied so that the rotor does not rotate and its speed becomes zero and full load current passes through the stator winding.
3. The slip is unity related to zero speed of rotor hence the load resistance becomes zero. Now, slowly increase the voltage in the stator winding so that the current reaches its rated value.
4. At this point, note down the readings of the voltmeter, wattmeter, and ammeter to know the values of voltage, power, and current. The test can be repeated at different stator voltages for the accurate value.
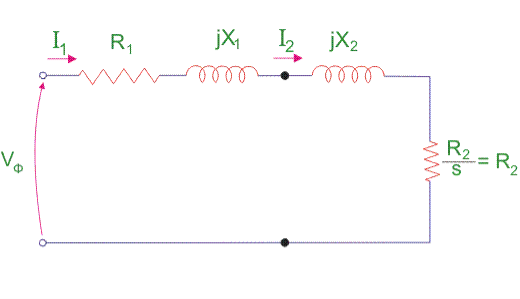
Fig. Block rotor test
5. Calculations of Blocked Rotor Test of Induction Motor
Resistance and Leakage Reactance Values
In blocked rotor test, core loss is very low due to the supply of low voltage and frictional loss is also negligible as the rotor is stationary, but stator copper losses and the rotor copper losses are reasonably high.
Let us take denote copper loss by W cu.
Therefore,

Where Wc = core loss

Where R01 = Motor winding of stator and rotor as per phase referred to the stator.
Thus,
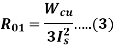
Now let us consider
Is = short circuit current
Vs = short circuit voltage
Z0 = short circuit impedance as referred to stator
Therefore,

X01 = Motor leakage reactance per phase referred to stator can be calculated as
Stator reactance X1 and rotor reactance per phase referred to as stator X2 is normally assumed equal.
Therefore,

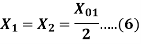
Similarly, stator resistance per phase R1 and rotor resistance per phase referred to stator R2 can be calculated as follows:
First, some suitable test is done on stator windings to find the value of R1 and then to find R2 subtract the R1 from R01

Short Circuit Current for Normal Supply Voltage
To calculate short circuit current Isc at normal voltage V of the stator, we must note short-circuit current Is and low voltage Vs applied to the stator winding.
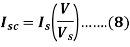
Key takeaways:
1. In the blocked rotor test, it should be kept in mind that the applied voltage on the stator terminals should be low otherwise normal voltage could damage the winding of the stator.
2. The testing of the induction motor is a little bit complex as the resultant value of leakage impedance may get affected by rotor position, rotor frequency, and magnetic dispersion of the leakage flux path.
3. To calculate short circuit current Isc at normal voltage V of the stator, we must note short-circuit current Is and low voltage Vs applied to the stator winding.
The efficiency of 3 phase induction motor:
Efficiency is defined as the ratio of output to that of input

Rotor efficiency of 3 phase induction motor

Gross mechanical power developed /rotor input

Three-phase induction motor efficiency

Three-phase induction motor efficiency

We classify starting methods for squirrel cage induction motor into two types based on voltage. The two types are:
1. Full voltage starting method
2. Reduced voltage method for starting squirrel cage induction motor.
Full Voltage Starting Method for Squirrel Cage Induction Motor
In this type, we have only one method of starting.
1. Direct on Line Starting Method
1. This method is also known as the DOL method for starting the three-phase squirrel cage induction motor. In this method, we directly switch the stator of the three-phase squirrel cage induction motor on to the supply mains.
2. The motor at the time of starting draws a very high starting current (about 5 to 7 times the full load current) for a very short duration.
3. The amount of current drawn by the motor depends upon its design and size. But such a high value of current does not harm the motor because of the rugged construction of the squirrel cage induction motor.
4. Such a high value of current causes sudden undesirable voltage drop in the supply voltage. A live example of this sudden drop of voltage is the dimming of the tube lights and bulbs in our homes at the instant of starting of refrigerator motor.
5. Now let us derive the expression for starting torque in terms of full load torque for the direct online starter. We have various quantities that involved in the expression for the starting torque are written below: We define Ts as starting torque
Tf as the full load torque
If as per phase rotor current at full load
Is as per phase rotor current at the time of starting
Sf as full load slip
Ss as starting slip
R2 as rotor resistance
Ws as the synchronous speed of the motor
Now we can directly write the expression for the torque of the induction motor as
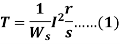
From the help of the above expression, we write the ratio of starting torque to full load torque as
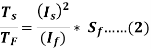
Here we have assumed that the rotor resistance is constant and it does not vary with the frequency of the rotor current.
Reduced voltage method for starting squirrel cage induction motor
In the reduced voltage method, we have three different types of starting method and these are written below:
1. Stator resistor starting method
2. Autotransformer staring method
3. Star delta starting method
Now let us discuss each of these methods in detail.
1. Stator Resistor Starting Method
Given below is the figure for the starting resistor method:
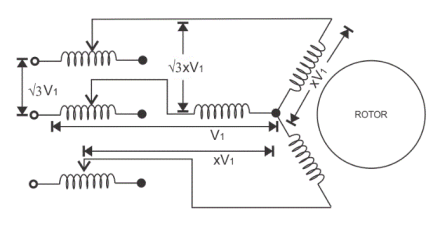
Fig. Stator resistor
1. In this method we add a resistor or a reactor in each phase as shown in the diagram (between the motor terminal and the supply mains). Thus by adding a resistor we can control the supply voltage.
2. Only a fraction of the voltage (x) of the supply voltage is applied at the time of starting of the induction motor. The value of x is always less than one.
3. Due to the drop in the voltage the starting torque also decreases. We will derive the expression for the starting torque in terms of the voltage fraction x to show the variation of the starting torque with the value of x.
4. As the motor speeds up the reactor or resistor is cut out from the circuit and finally, the resistors are short-circuited when the motor reaches its operating speed.
5. Now let us derive the expression for starting torque in terms of full load torque for the stator resistor starting method. We have various quantities that involved in the expression for the starting torque are written below: we define Ts as starting torque
Tf as the full load torque
If as per phase rotor current at full load
Is as per phase rotor current at the time of starting
Sf as full load slip
Ss as starting slip
R2 as rotor resistance
Ws as the synchronous speed of the motor
Now we can directly write the expression for the torque of the induction motor as
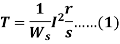
From the help of the above expression, we write the ratio of starting torque to full load torque as
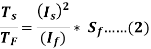
Here we have assumed that the rotor resistance is constant and it does not vary with the frequency of the rotor current. From the above equation, we can have the expression for the starting torque in terms of the full load torque.
6. Now at the time of starting the per phase voltage is reduced to xV1, the per phase starting current is also reduced to xIs. On substituting the value of Is as xIs in the equation We have
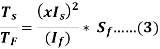
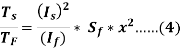
7. This shows the variation of the starting torque with the value of x. Now there are some considerations regarding this method. If we add a series resistor then the energy losses are increased so it’s better to use a series reactor in place of a resistor because it is more effective in reducing the voltage however series reactor is more costly than the series resistance.
2. Auto Transformer Starting Method
1. As the name suggests in this method we connect the auto transformer in between the three-phase power supply and the induction motor as shown in the given diagram:
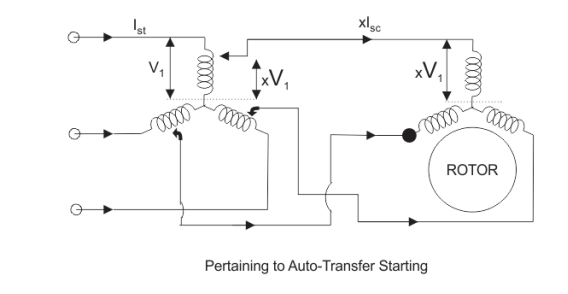
Fig. Stating of autotransformer
2. The autotransformer is a step-down transformer hence it reduces the per phase supply voltage from V1 to xV1. The reduction in voltage reduces current from Is to xIs.
3. After the motor reaches its normal operating speed, the autotransformer is disconnected and then full line voltage is applied.
3. Now let us derive the expression for starting torque in terms of full load torque for the autotransformer starting method. We have various quantities that involved in the expression for the starting torque are written below:
We define Ts as starting torque
Tf as the full load torque
If as per phase rotor current at full load
Is as per phase rotor current at the time of starting
Sf as full load slip
Ss as starting slip
R2 as rotor resistance
Ws as the synchronous speed of the motor
Now we can directly write the expression for the torque of the induction motor as
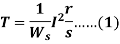
From the help of the above expression, we write the ratio of starting torque to full load torque as
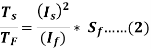
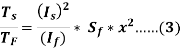
4. Here we have assumed that the rotor resistance is constant and it does not vary with the frequency of the rotor current. From the above equation, we can have the expression for the starting torque in terms of the full load torque.
5. Now at the time of starting the per phase voltage is reduced to xV1, the per phase starting current is also reduced to xIs. On substituting the value of Is as xIs in equation 1. We have
This shows the variation of the starting torque with the value of x.
3. Star-Delta Starting Method
The connection diagram is shown below for the star-delta method,
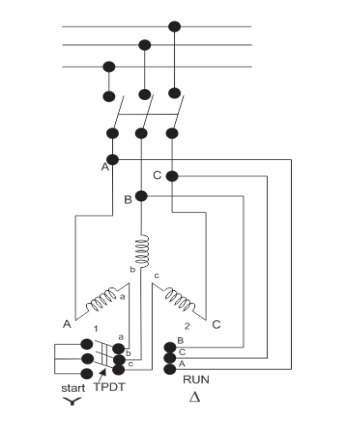
Fig. Star delta connection
1. This method is used for the motors designed to operate in delta connected winding. The terminals are marked for the phases of the stator are shown above. Now let us see this method works.
2.The stator phases are first connected to the star with the help of a triple pole double throw switch (TPDT switch) in the diagram the position is marked as 1 then after this when the steady-state speed is reached the switch is thrown to position 2 as shown in the above diagram.
3. Now, let analyze the working of the above circuit. In the first position, the terminals of the motor are short-circuited and in the second position from the diagram the terminal a, b and c are respectively connected to B, C, and A.
4. Now let us derive the expression for starting torque in terms of full load torque for the star-delta starting method. We have various quantities that involved in the expression for the starting torque are written below
Tf as the full load torque
Ts as starting torque
If as per phase rotor current at full load
Is as per phase rotor current at the time of starting
Sf as full load slip
Ss as starting slip
R2 as rotor resistance
Ws as the synchronous speed of the motor
Now we can directly write the expression for the torque of the induction motor as
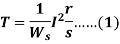
From the help of the above expression, we write the ratio of starting torque to full load torque as
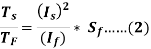
5. Here we have assumed that the rotor resistance is constant and it does not vary with the frequency of the rotor current. Let us assume the line voltage to be Vl then the per phase starting current when connected in star position is Iss which is given by
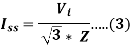
When the stator is in delta connected position we have starting current

From the above equation we have
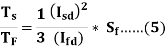
6. This shows that the reduced voltage method has the advantage of reducing the starting current but the disadvantage is that all these methods of reduced voltage cause the objectionable reduction in the starting torque.
Key takeaways:
1. In DOL the amount of current drawn by the motor depends upon its design and size
2. In the stator resistor starting method Due to the drop in the voltage the starting torque also decreases.
3. In autotransformer starting method. After the motor reaches its normal operating speed, the autotransformer is disconnected and then full line voltage is applied.
4.star delta starting method is used for the motors designed to operate in delta connected winding.
1. When it comes to controlling an electric machine by electric drivers braking is a very important term because it helps to decrease the speed of the motor according to will and necessity. The braking of induction motors can be classified mainly into three types
Regenerative braking.
1. Plugging or reverse voltage braking
2. Dynamic braking which can be further classified as
3. AC dynamic braking
4. Self-excited braking using capacitors
5. DC dynamic braking
6. Zero sequence braking
To explain that regeneration braking for induction motor, we can take help of the equation
1. Here, θs is the phase angle between the stator voltage and stator current, the simple words whenever this phase angle exceeds 90o (i.e θs>90o) regenerative braking can take place.
2. To explain this more clearly and easily we can say that whenever the speed of the rotor exceeds synchronous speed, regeneration braking occurs.
3. That is because whenever the rotor rotates at a speed more than synchronous speed there is a reverse field occurs which opposes the normal rotation of the motor and therefore braking takes place.
4. Main disadvantage of this type of braking is that the speed of the motor has to exceed synchronous speed which may not be possible every time. To acquire regenerative braking at a lower speed than synchronous speed, a variable frequency source can be used.
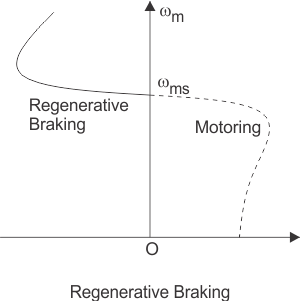
Fig. Braking graph
6. Plugging of induction motors is done by interchanging any two of the supply terminals. When the terminals have reversed the operation of the machine changes from motoring to plugging.
7. From a technical point of view and for better understanding it can be said that the slip changes from‘s’ to (2-s), which indicates that due to reversal of the terminals the torque also changes its direction and braking occurs.
8. The first classification of dynamic braking of induction motors is AC dynamic braking any one of the supply phases is disconnected from the supply and then it is either kept open or connected with the other phase.
9. The first type is known as two lead connections and the second one is known as three lead connections.
10. To understand this braking method we can assume the system to be a single-phase system. Now the motor can be considered to be fed by positive and negative sequence voltages. That’s why when the rotor resistance is high the net torque is negative and braking can be acquired.
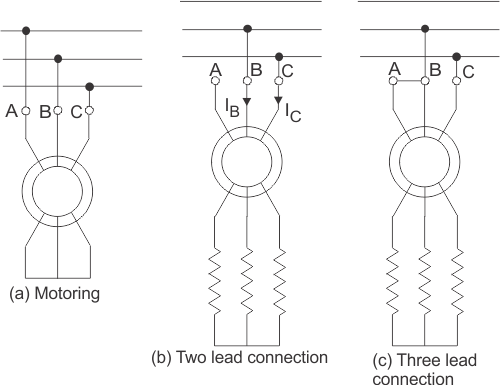
Fig. Braking systems in dynamic induction
11. Sometimes capacitors are kept permanent by connected across the supply terminals of the motor. This is called self-excited braking using capacitors of induction motors.
12. This type of braking works mainly by the property of the capacitors to store energy. Whenever the motor is disconnected from the supply the motor starts to work as a self-excited induction generator, the power comes from the capacitors connected across the terminals.
13. The values of the capacitor are so chosen that they are sufficient to make the motor work as an induction generator after being disconnected from the supply. When the motor works as an induction generator the produced torque opposes the normal rotation of the motor and hence braking takes place.
Speed control of induction motor
Speed control of induction motors can be done in six methods which are
1. Pole changing
2. Stator voltage control
3. Supply frequency control
4. Eddy current coupling
5. Rotor resistance control
1. We know that the speed of the induction motor is inversely proportional to several poles. So it is possible to increase or decrease the speed of the induction motor if the number of the poles is decreased or increased respectively.
2. The motor in which the provision of changing the number of poles is present, they are called ‘pole changing motor’ or ‘multi-speed motor’.
3. Another method of controlling the speed of induction motor drives is stator voltage control. Stator voltage is directly responsible for the rotating speed of the rotor.
4. Torque is proportional to voltage squared and the current is proportional to the voltage. So, if the stator voltage is reduced the speed reduces, and similarly if the stator voltage is increased the speed also increases.
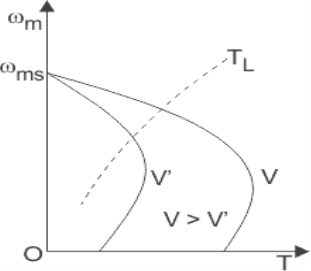
Fig. Speed control graph
5. The speed of an induction motor is proportional to the product of the supply frequency and air gap flux.
6. But as there is a chance of magnetic saturation while decreasing the supply frequency, that’s why not only the frequency but the v/f (i.e the ratio of supply voltage and frequency) is controlled and this ratio is tried to be kept constant. And if the speed is needed to be changed the ratio of v/f is changed accordingly.
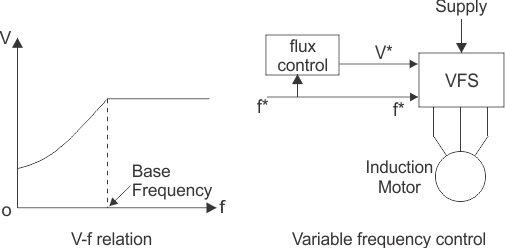
Fig. Voltage and frequency relation graph
7. The eddy current speed control method is done by placing an eddy current clutch between an induction motor is running at a fixed speed and the variable speed load. Now, what is this eddy current clutch?
8. It is nothing but an induction motor drive in which both the stator and the rotor are allowed to rotate. The rotor is coupled with the main induction motor.
9. When eddy currents are produced in the rotor drum, their interaction with the stator field and torque is produced which rotates the main motor. By controlling the DC current through the stator winding the speed of the motor can be controlled.
10. Depending on the rotor resistance, the speed of the rotor falls or increases. The variation of speed-torque characteristics with respect to change in rotor resistance is shown in the figures below.
11.This speed controlling method is better than many other methods because of its low cost
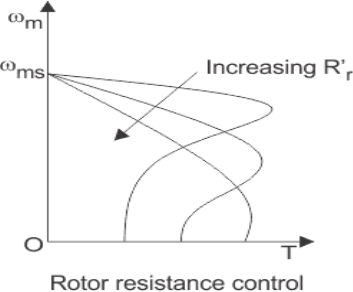
Fig. Resistance control graph
Key takeaways:
1. Torque is proportional to voltage squared and the current is proportional to the voltage. So, if the stator voltage is reduced the speed reduces, and similarly if the stator voltage is increased the speed also increases.
2. The speed of an induction motor is proportional to the product of the supply frequency and air gap flux.
3. By controlling the DC current through the stator winding the speed of the motor can be controlled.
It is possible to produce a variable rotor resistance by the use of deep rotor bars or double cage rotors. The basic concept is shown below. Figure a shows a current flowing through the upper part of a deep rotor bar. Since current flowing in that area is tightly coupled to the stator, the leakage inductance is small for the region. Figure b shows current flowing deeper in the bar. Here, the leakage inductance is higher. Since all parts of the rotor bars are in parallel electrically, the bar essentially represents a series of parallel electric circuits, the upper ones having a smaller induuctances nd the lower ones having a larger incuctance.
At low slip, the rotor’s frequency is very small, and the reactances of all the parallel paths through the bar are small compared to their resistances. The impedances of all parts of the bar are approximately equal, so current flows through all parts of the bar equally. The resulting large cross sectional area makes the rotor resistance quite small resulting in good efficiency at low slip. At high slip the reactance are large compared to the resistance in the bar, so all the current is forced to flow in the low resistance is higher than before. With a high rotor resistance at starting conditions, the starting torque is relatively higher and the starting current is relatively lower than in a class A design.
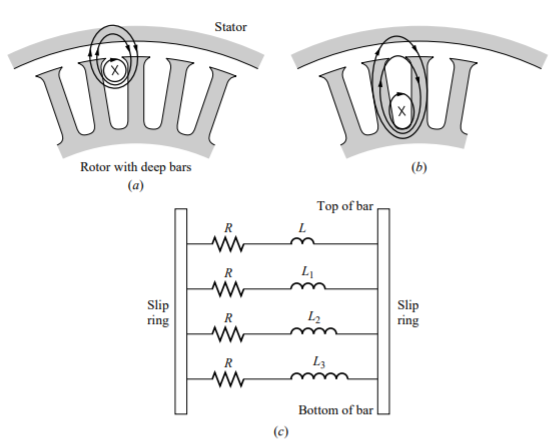
The cross sectional view of a double cage rotor is shown in figure c. It consists of a large, low resistance set of bars buried deeply in the rotor and a small, high resistance set of bars set at the rotor surface. It is similar to the deep bar rotor,except that the difference between low slip and high slip operation is even more exaggerated. At starting conditions, only the small bar is effective, and the rotor resistance is quite high. This high resistance results in a large starting torque. Howeever, at normal operating speeds, both bars are effective, and the resistance is almost as low as in a deep bar rotor. Double cage rotors of this sort are used to produce NEMA class B and class C characteristics.
Doblu cage rotors have disadvantage that they are more expensive than the other types of squirrel cage rotors, buut they are cheaper than wound rotor designs. They allow some of the best features possible with wound rotor motors at a lower cost and without the need of maintaining slip rings and brushes.
Sometimes, squirrel cage induction motor exhibits a tendency to run at low speed. This phenomenon is called as crawling of an induction motor. This action is due to the fact that, flux wave produced by a stator winding is not purely sine wave. Instead, it is a complex wave consisting a fundamental wave and odd harmonics like 3rd, 5th, 7th etc. The fundamental wave revolves synchronously at synchronous speed Ns whereas 3rd, 5th, 7th harmonics may rotate in forward or backward direction at Ns/3, Ns/5, Ns/7 speed respectively. Heence, harmonic torques are also develppoed in addition with fundamental torque.
3rd harmonics are absent in a balanced 3-phase system. Hence, 3rd harmonics do not produce rotating field and torque. The total motor torque now consist three components as:
(I) The fundamental torque with synchronous speed Ns
(II) 5th harmonic torque with synchronos speed Ns/5
(III) 7th harmonic torque with synchronous speed Ns/7
Now, 5the harmonic currents will have phase difference of
5x120=6000=2x360-120=-1200
Hence the revolving speed set up will be in reverse direction with speed Ns/5. The small amount of 5th harmonic torque produces breaking action and can be neglected. The 7th harmonic current will have phase difference of
7x120=8400=2x360+120=+1200
Hence, they will set up rotating field in forward direction with synchronous speed of Ns/7. If we neglect all the higher harmonics, the resultant torque will be equal to sum of fundamental torque and 7th harmonics torque. 7th harmonic torque reaches its maximum positive value just before 1/7th of Ns. If the mechanical load on the shaft involves constant load torque, the torque developed by the motor may fall below this load torque. In this case, motor will not accelerate up to its normal speed, but it will run at a speed which is mearly 1/7th of its normal speed as shown below. This phenomenon is caleed as crawling of induction motor.
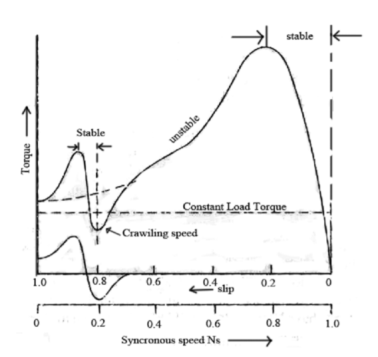
Cogging (Magnetic Locking or Teeth Locking) of Induction Motor
Sometimes, the rotor of a squirrel cage induction motor refuses to start at all, particularly if the supply voltage is low. This happens especially when number of rotor teeth is equal to number of stator teeth, because of magnetic loa=cking between the stator teeth and the rotor teeth. When the rotor teeth and stator teeth face each other, the reluctance of the magnetic path is minimum that is why the rotor tends to remain fixed. This phenomenon is called as cogging or magnetic locking of induction motor.
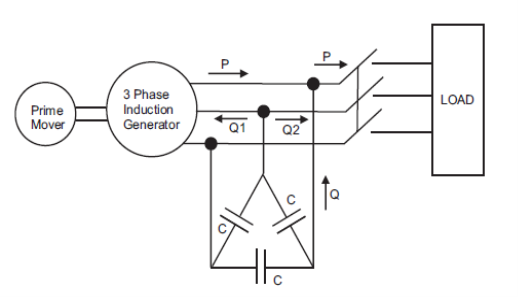
Fig. Generator diagram
Induction machines are sometimes used as a generator. These are known as induction generators or asynchronous generators. So under what conditions an induction machine will behave as an induction generator?
An induction machine will behave as an induction generator when:
1. Slip becomes negative due to this the rotor current and rotor emf attains negative value.
The prime mover torque becomes the opposite of electric torque.
2. Now let us discuss how we can achieve these conditions. Suppose that an induction machine is coupled with the prime mover whose speed can be controlled.
3. If the speed of the prime mover is increased such that the slip becomes negative (i.e. speed of the prime mover becomes greater than the synchronous speed).
4. Due to this, all the conditions that we have mentioned above will become fulfilled and the machine will behave like an induction generator. Now if the speed of the prime mover is further increased such that it exceeds the negative maximum value of the torque produced then the generating efficiency of the generator vanishes.
5. Clearly, the speed of the induction generator during the whole operation is not synchronous, therefore the induction generator is also called a synchronous generator.
6.An induction generator is not a self-excited machine. Therefore To develop the rotating magnetic field, requires magnetizing current and reactive power. The induction generator obtains its magnetizing current and reactive power from various sources like the supply mains or it may be another synchronous generator.
Key takeaways:
1. Slip becomes negative due to this the rotor current and rotor emf attains negative value. The prime mover torque becomes the opposite of electric torque.
2. To develop the rotating magnetic field, requires magnetizing current and reactive power
Applications of Induction generators
Induction generators are used principally with alternative energy sources such as windmills or wind energy recovery systems for large scale power generation. They are also used to supply additional power to a load in a remote area that is being supplied by a weak transmission line.
Example
A 10 hp, 1760 rpm, 440V, three-phase induction motor operates as an asynchronous generator. The full load current of the motor is 10 A and the full load power factor is 0.8. Determine the required capacitors per phase if capacitors are connected in delta.
Answer-
Apparent power



Active power



Reactive power


For a machine to run as an asynchronous generator, capacitor bank must supply a minimum VAR per phase
Capacitive current

Capacitive reactance per phase
Minimum capacitance per phase
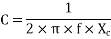
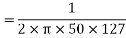

If the load also absorbs reactive power, the capacitor bank must be increased in size to compensate.
Prime mover speed should be used to generate a frequency of 50 Hertz.
CASE STUDY
Driver Circuit for Stepper Motor
Steppers are special motors that do not have any commutator to reverse flow of the windings current. Stepper motors are the best alternatives for applications that high-accuracy motions are required such as CNC, printers and so on. Because of the simple winding and control system, the main applications of these motors are the motion controls. On the basis of the motor structure and operation, stepper motors are divided into three types: permanent magnet (PM), variable reluctance (VR) and hybrids[7]. There is special procedure for driving each of them. In general, stepper motors are driving in full-, half- and micro-step modes. In full-step mode, to change rotor position completely, activation and deactivation signals are fed to the windings alternatively in each cycle.
In half-step, each winding can stay activated for more than on cycle. Therefore, the rotor motion is half of the full-step and the motor resolution is two times more. Micro-step driving is another alternative for motor motion that has more accuracy and continuous motion. Two main advantage of micro-step mode is the possibility for rotor to move between full and half modes and to eliminate irregular characteristics of motor torque. Stepper motor driver circuit implementation process for all three modes is
Presented. For design testing, appropriate driving signal patterns are generated using PC parallel port. Torque tests are applied to the driver circuit and experimental results extracted. As obtained, using micro-step driving, stepper motor rotates more continuously. Also, irregular torque values (appeared like holes in torque curve at resonance frequencies) are eliminated.
Control Circuit
Generally, control and driving techniques for stepper motors are categorized in open- and close-loop modes (here, open-loop mode is considered). In stepper motors, driving is done by activation and deactivation of various windings. In general stepper motor driver circuit is divided into two main parts: signaling and switching [8]. Signaling part is for generating the consequent and regular pulses (patterns) for motor driver. The switching part turns the control switches on and off according to the generated patterns. A four-phase stepper motor
Driver has two main parts as follows: control signal sequencer and motor driver
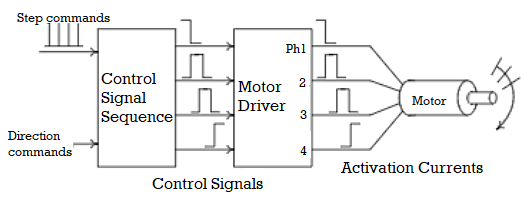
Fig. Driver Circuit
According to the nature of the windings, the current and therefore the switch state could not change suddenly except that infinite opposite voltage is applied to the winding. When the control switch of the winding is closed, the winding current increased gradually. Thus, when its state change (the switch opened), large voltage is applied to the winding. This may damage the control switches if there is no care about it. There are two main strategies to solve these problems: using diode or capacitance in parallel with the
Winding. There are several driving techniques for every type of the stepper motors. Consider a bipolar H Bridge hybrid motor, as shown in Fig2. Reversing the magnet field direction, one can change the winding current flow. Achieving this, one can arrange the control switches like H letter (called H-Bridge) and put the winding between them.
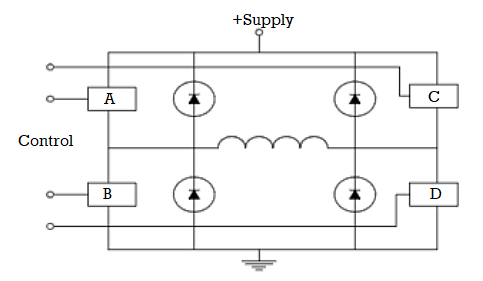
Fig. Bridge Hybrid Model
Because of four control switches, H-Bridge can have 16 different modes of operations. Some of
Them are not used generally. Some of the practical operation modes are as follows
- Forward: only A and D switches are closed.
- Reverse: only B and C switches are closed.
- Fast Decay: All switches are closed and the winding current decreased through the power
- Supply and diodes path. This mode doesn’t damage the winding and therefore the rotor can move freely without any resistance.
- Slow Decay: In this mode, the current is passed through the motor winding rotationally without any resistance. Therefore, if a few current remains in winding, this operation caused the current dissipated and the rotor stopped slowly.
It is possible that activation of control switches in H-Bridges caused short-circuit between power supplies (i.e. closing switches A and B). In these cases, usually logic gates used to eliminate short-circuit effects. Fig 3 shows the sample circuit where this technique is applied.
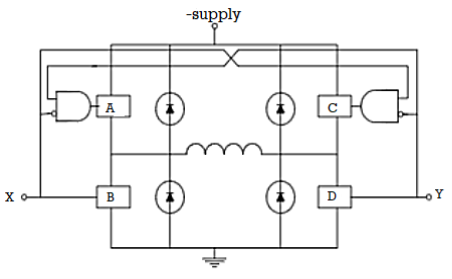
Fig. SC elimination from H bridge
As shown above, all of the operation modes can be accessible only with a few numbers of signals.
This is so practical when we use microcontrollers or microprocessors for control signal (pattern) generation (i.e. X and Y signals on the above).
Micro-step driving circuit
In micro-step mode[7], the resolution (number of steps per round) is increased. The motor used pass 1.8 degree per rotation and has resolution equals to 200 steps. After micro-step driving, the target becomes 800 steps per rotation (4 micro-steps per each step). After driver implementation for half- and full-step
Modes, several parts should be changed and optimized for micro-step driving. One of the main
Parts is the reference voltage (Vref). Another optimization is to control two phase of the motor independently. For this purpose, driver circuit must be changed to be able to control the winding
Current independently to the portion of the final phase current. For this, one can control the current
Of the motor phases to be able to move between two full steps more. According to this, digital to
Analog converter (DAC) and operational amplifiers (OpAmp) are used to control the reference voltage
Of the motor. Inputs to the ADC will determined the signal levels and the bit number can perform
More accurately. Two L297 devices are used to control the L298 phases separately. These devices
Should be synchronized by each other. The inhibit output signals of each L297 (INH) control
Corresponding enable (EN) lines of the L298 (ENA and ENB).
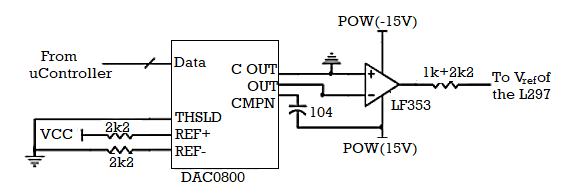
Fig. Micro-step driving circuit
References:
1. A. E. Fitzgerald and C. Kingsley, "Electric Machinery”, McGraw Hill Education,2013.
2. M. G. Say, “Performance and design of AC machines”, CBS Publishers,2002.
3. P. S. Bimbhra, “Electrical Machinery”, Khanna Publishers,2011.
4. I. J. Nagrath and D. P. Kothari, “Electric Machines”, McGraw Hill Education,2010.
5. A. S. Langsdorf, “Alternating current machines”, McGraw Hill Education,1984.
6. P. C. Sen, “Principles of Electric Machines and Power Electronics”, John Wiley & Sons,2007.