Unit - 1
Introduction
The regularity with which atoms or ions are placed regarding one another can be used to classify solid materials. A crystalline substance is one in which the atoms are arranged in a repeating or periodic pattern over vast atomic distances; that is, long-range order exists, allowing the atoms to solidify in a repetitive three-dimensional pattern in which each atom is bound to its nearest neighbours. Under normal solidification conditions, all metals, many ceramic materials, and some polymers produce crystalline forms.
In a solid, an atom or an ion has nearly the same number and type of nearest neighbours in both the crystalline and non-crystalline versions of the solid. The non-crystalline structure, on the other hand, lacks the crystalline state's long-range regularity. Any number of integral lattice translations in a crystal would take us from one atom at one lattice point to another identical atom at another lattice point. In the non-crystalline state, however, this is not the case. Because diffraction effects are based on long-range periodicity, non-crystalline substances do not produce sharp diffraction patterns like crystals.
Non crystalline structures are favoured by several variables. One-dimensional chain molecules and two-dimensional sheet molecules are generated when primary bonds do not stretch in all directions. Secondary bonding forces must assist such units in forming a three-dimensional crystal.
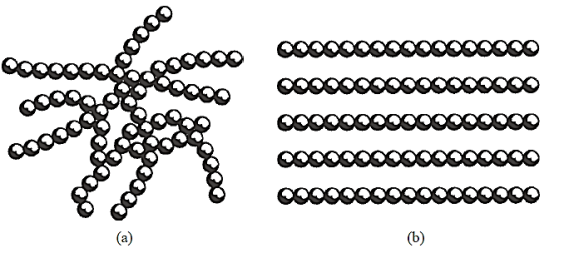
The crystalline state's free energy is always smaller than the non-crystalline states. The inclination to crystallise will be substantial only when the free energy difference between the two states is large. Some materials have an open network structure of atoms, with minimal change in free energy between an orderly and disorderly arrangement of the units. The inclination to crystallise will be weak in such instances.
The rate of cooling from the liquid state is a third element that favours the creation of non-crystalline structures. Along the path of transition from the liquid to the crystalline state, kinetic barriers occur. Slow cooling rates allow crystallisation to occur, whereas fast cooling rates may prevent crystallisation entirely. Depending on the magnitude of the kinetic barrier, the rate of cooling that is considered slow or fast will vary greatly for different materials.
In contrast to the non-crystalline material, which gradually softens across a range of temperatures, the crystal has a fast-melting point. The atoms or molecules in a crystal are more closely packed because of the regularity of the arrangement. As a result, the crystalline form is denser than the non-crystalline form. The average strength of the secondary bonds present tends to grow when the crystal is packed closer together.
Depending on the level, we can classify the structure of materials as:
- Macrostructure
A material's macrostructure is evaluated with the naked eye or under low magnification. The atomic groupings of a crystalline material's internal symmetry may be reflected in the exterior form of a crystal-like quartz. The repeated polishing and etching action of the human hand and sweat can make large individual crystals of a crystalline substance visible to the naked eye, as in a brass doorknob.
- Microstructure
The term "microstructure" refers to the structure seen using an optical microscope. This microscope can magnify a structure up to 1500 times linearly without losing precise resolution. The human eye's resolution limit is around 0.1 mm, which means that the eye can only discern two lines as independent lines if their separation is greater than 0.1 mm. The optical microscope can resolve details down to roughly 0.1µm.
- Substructure
The structure acquired by using a microscope with a significantly higher magnification and resolution than an optical microscope is referred to as substructure. A magnification of 1 000 000 times linear is feasible in an electron microscope. Because electrons have a shorter wavelength than visible light, their resolving power improves in tandem, allowing the electron microscope to see considerably finer details. On very small particles or crystal flaws such as dislocations, we can gain a lot of new information.
- Crystal structure
The atomic arrangement within a crystal is described by crystal structure. In most cases, describing the arrangement of a few atoms within a unit cell is adequate. The crystal is made up of a vast number of unit cells that form regular patterns in space. X-ray diffraction is the most used method for identifying crystal structure.
- Electronic structure
The electrons in the outermost orbitals of individual atoms that make up a solid's electronic structure are commonly referred to as the solid's electronic structure. When it comes to establishing the electronic structure, spectroscopic techniques are extremely beneficial.
- Nuclear structure
Nuclear spectroscopy techniques such as nuclear magnetic resonance (NMR) and Mossbauer studies are used to investigate nuclear structure.
Key Takeaway:
A crystalline substance is one in which the atoms are arranged in a repeating or periodic pattern over vast atomic distances; that is, long-range order exists, allowing the atoms to solidify in a repetitive three-dimensional pattern in which each atom is bound to its nearest neighbours.
The non-crystalline structure, lacks the crystalline state's long-range regularity
We can classify them in three broad groups according to their nature:
- Metals and alloys
- Ceramics and glasses
- Organic polymers
Metals are well-known items with a distinct look; they may change shape permanently and have excellent thermal and electrical conductivity. An alloy is a mixture of several metals. Ceramics and glasses are brittle non-metallic inorganic materials with strong thermal and electrical insulating qualities. Organic polymers are relatively inert and light, with a high degree of flexibility in general.
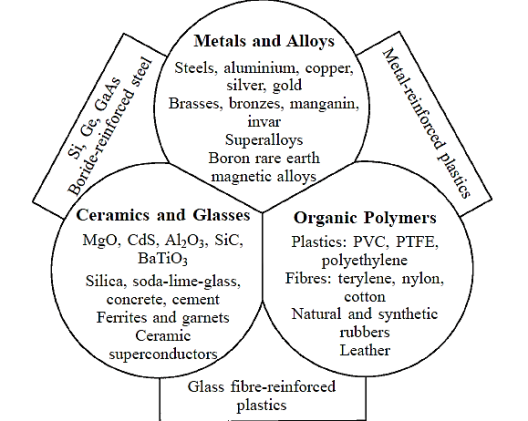
Metals:
This set of materials contains one or more metallic elements (e.g., iron, aluminium, copper, titanium, gold, and nickel) as well as non-metallic elements (e.g., carbon, nitrogen, and oxygen) in modest proportions. Metals and their alloys have very ordered atom arrangements and are relatively dense in compared to ceramics and polymers. Mechanically, these materials are quite stiff and strong, but they are also ductile (i.e., capable of substantial quantities of deformation without fracture) and fracture resistant, which explains their widespread usage in structural applications.
Nonlocalized electrons, or electrons that are not bound to specific atoms, are abundant in metallic materials. These electrons are directly responsible for many of the properties of metals. Metals, for example, are excellent conductors of electricity and heat and are not visible light translucent; a polished metal surface has a shiny aspect. Furthermore, several of the metals (e.g., Fe, Co, and Ni) have magnetic properties that are desirable.
Ceramics:
Ceramics are mixtures of metallic and non-metallic materials, with oxides, nitrides, and carbides being the most common. Aluminium oxide (or alumina, Al2O3), silicon dioxide (or silica, SiO2), silicon carbide (SiC), silicon nitride (Si3N4), and, in addition, what some refer to as classic ceramics—those made of clay minerals (i.e., porcelain), cement, and glass—are all typical ceramic materials. Ceramic materials are relatively stiff and robust mechanically—their stiffnesses and strengths are equivalent to those of metals.
Furthermore, they are usually extremely difficult. Ceramics have long been known for their exceptional brittleness (lack of elasticity) and susceptibility to fracture. Newer ceramics, on the other hand, are being developed to have better fracture resistance; these materials are utilised in cookware, cutlery, and even vehicle engine parts. Ceramic materials are also more resistant to high temperatures and severe environments than metals and polymers because they are insulative to the flow of heat and electricity (i.e., have low electrical conductivities).
Ceramics can be transparent, translucent, or opaque in terms of optical properties, and some oxide ceramics (e.g., Fe3O4) have magnetic properties.
Polymers:
Polymers include things like plastic and rubber. Many of them are organic molecules made up of carbon, hydrogen, and other non-metallic components in their chemical makeup (i.e., O, N, and Si). They also have very massive molecular structures, which are frequently chainlike in character and feature a carbon atom backbone. Polyethylene (PE), nylon, poly(vinyl chloride) (PVC), polycarbonate (PC), polystyrene (PS), and silicone rubber are some of the most prevalent and well-known polymers.
These materials usually have low densities, and their mechanical properties differ from those of metallic and ceramic materials—they are not as stiff or robust as these other material kinds. However, because of their low densities, their stiffnesses and strengths are often equivalent to metals and ceramics on a per-mass basis. Furthermore, many of the polymers are ductile and flexible (i.e., plastic), making them easy to mould into complicated shapes.
Chemically, they are relatively inert and unreactive in a wide variety of conditions. The polymers' tendency to soften and/or breakdown at low temperatures is one of their significant drawbacks, which limits their application in several cases. They are also nonmagnetic and have poor electrical conductivities.
Key Takeaway:
Metals are well-known items with a distinct look; they may change shape permanently and have excellent thermal and electrical conductivity. An alloy is a mixture of several metals. Ceramics and glasses are brittle non-metallic inorganic materials with strong thermal and electrical insulating qualities. Organic polymers are relatively inert and light, with a high degree of flexibility in general.
Ionic Bond
The easiest to define and see is ionic bonding. It is always found in compounds that contain both metallic and non-metallic elements, as well as elements from the periodic table's horizontal axis. The valence electrons of metallic atoms are easily given up to non-metallic atoms. All of the atoms in the process gain stable or inert gas configurations as well as an electrical charge, transforming them into ions.
The prototypical ionic substance is sodium chloride (NaCl). A transfer of a sodium atom's one valence 3s electron to a chlorine atom can give it the electron structure of neon (and a net single positive charge). The chlorine ion has a net negative charge and an electron configuration that is identical to that of argon after such a transfer. All of the sodium and chlorine in sodium chloride exist as ions.
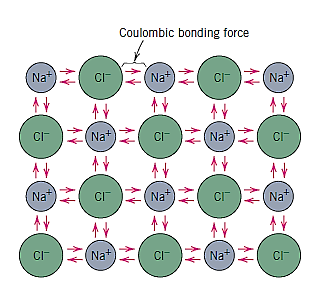
The attractive bonding forces are coulombic, which means that positive and negative ions are attracted to one another based on their net electrical charge.
Nondirectional ionic bonding means that the bond's magnitude is the same in all directions surrounding an ion. In order for ionic materials to be stable, all positively charged ions must have negatively charged ions as nearest neighbours in a three-dimensional scheme, and vice versa. Ionic bonding is the most common type of bonding in ceramic materials.
Key Takeaway:
It is always found in compounds that contain both metallic and non-metallic elements, as well as elements from the periodic table's horizontal axis. The valence electrons of metallic atoms are easily given up to non-metallic atoms
Covalent Bond
In covalent bonding, stable electron setups are expected by the sharing of electrons between neighbouring atoms. Two atoms that are covalently fortified will each contribute no less than one electron to the bond, and the mutual electrons might be considered to have a place with both particles. Covalent holding is schematically delineated in Figure 2.9 for an atom of methane (CH4). The carbon particle has four valence electrons, though each of the four hydrogen molecules has a solitary valence electron. Every hydrogen iota can obtain a helium electron arrangement (two 1s valence electrons) when the carbon molecule offers with it one electron. Carbon now has four extra shared electrons, one from every hydrogen, for an aggregate of eight valence electrons, and the electron structure of neon. The covalent bond is directional; that is, it is between particular particles and may exist just in the course between one iota and another that takes part in the electron sharing.
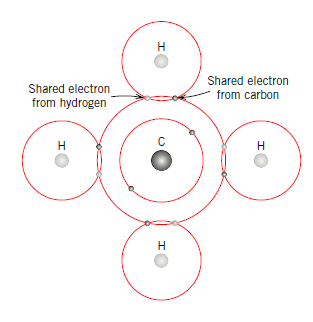
Hence, we can summarize covalent bonding that in this type of bonding electrons are shared between the atoms, to soak the valency. The most straightforward case is the H2 particle, where the electrons invest more energy in the middle of the cores of two iotas than outside, along these lines delivering holding. Covalent bonds are stereo particular, i.e., every bond is between a particular match of particles, which share a couple of electrons (of inverse attractive twists). Regularly, covalent bonds are exceptionally solid, and directional in nature. The hardness of precious stone is an aftereffect of the way that every carbon molecule is covalently reinforced with four neighbouring particles, and every neighbour is fortified with an equivalent number of atoms to shape an inflexible three-dimensional structure.
Key Takeaway:
In covalent bonding, stable electron setups are expected by the sharing of electrons between neighbouring atoms. Two atoms that are covalently fortified will each contribute no less than one electron to the bond, and the mutual electrons might be considered to have a place with both particles
Metallic Bond
Metals and their alloys have metallic bonding, which is the final main bonding type. A simple model has been devised that comes pretty close to describing the bonding system. One, two, or three valence electrons are present in metallic materials. These valence electrons are not bonded to any specific atom in the solid in this scenario, and they are more or less free to float across the metal. They can be conceived of as constituting a "sea of electrons" or a "electron cloud," or as belonging to the metal as a whole.
Ion cores are formed by the leftover non valence electrons and atomic nuclei, and they have a net positive charge equal to the total valence electron charge per atom. The positively charged ion cores are shielded by free electrons from mutually repulsive electrostatic forces that they would otherwise exert on one another, resulting in a nondirectional metallic bond. These free electrons also serve as a "glue" that holds the ion cores together.
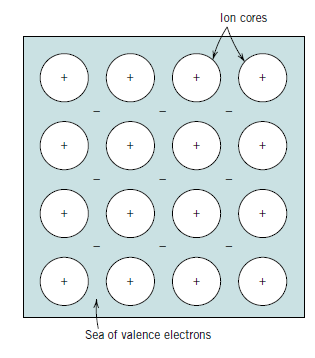
Ion cores are formed by the leftover non valence electrons and atomic nuclei, and they have a net positive charge equal to the total valence electron charge per atom. The positively charged ion cores are shielded by free electrons from mutually repulsive electrostatic forces that they would otherwise exert on one another, resulting in a nondirectional metallic bond. These free electrons also serve as a "glue" that holds the ion cores together.
Ionically and covalently bound materials, on the other hand, are often electrical and thermal insulators due to the lack of large amounts of free electrons.
Key Takeaway:
One, two, or three valence electrons are present in metallic materials. These valence electrons are not bonded to any specific atom in the solid in this scenario, and they are more or less free to float across the metal.
Ion cores are formed by the leftover non valence electrons and atomic nuclei, and they have a net positive charge equal to the total valence electron charge per atom. The positively charged ion cores are shielded by free electrons from mutually repulsive electrostatic forces that they would otherwise exert on one another, resulting in a nondirectional metallic bond.
References:
- Material Science and Engineering by V. Raghavan
- Material Science and Engineering by William D. Callister
- Material Science by Prof. Satish V. Kailas (Associate Professor, Dept. Of Mechanical Engineering, Indian Institute of Science, Bangalore – 560012) NPTEL