Unit - 1
Introduction of Wireless Channel
First-generation analog cellular telephone
- First generation cellular and cordless telephone networks are based on analog circuit switching technology. The first 1Gmobile phone was introduced in the USA in 1980. FDMA was the multiple access technique used and worked mainly in the 800-900 MHz frequency bands. The 1Gmobile phone had only voice facility.
- Examples of 1G systems are AMPS (Advanced mobile phone service) and TACS (total access communication systems)
- The limitations of 1 Gare:
- Supports Speech only
- Low traffic capacity
- Unreliable handover
- Long call setup time
- Frequent call drops
- Inefficient bandwidth usage
- Poor battery life
- Poor voice quality
- Large size of handset
- Allowed users to make voice calls within a country only
- A typical example of a cellular telephone system is the Advance Mobile Phone Services (AMPS) system used in the United States. In 1971, Bell Telephone Laboratories in Murry Hill, New Jersey, proposed the cellular telephone concept as the Advanced Mobile Telephone System (AMPS). AMPS is a standard cellular telephone service (CTS) initially placed into operation on October 13, 1983, by Illinois Bell that incorporated several large cell areas to cover approximately 2100squaremilesin the Chicago area.
- Basically, all first generation systems use the transport architecture shown in the figure
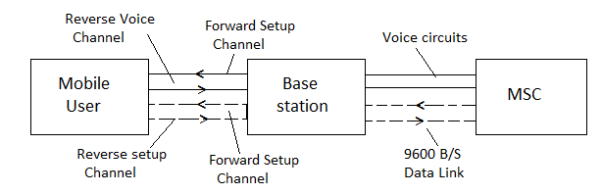
Fig 1 Architecture
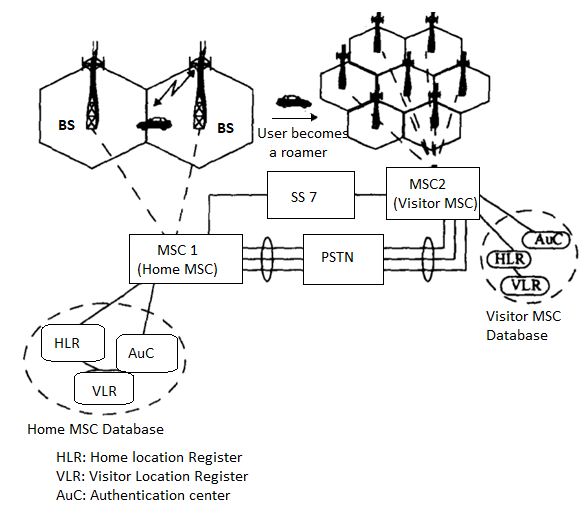
Fig 2 Block Diagram of First-Generation Cellular System
The block diagram of a first-generation cellular telephone network is shown in the figure. The system control for each market resides in the MSC which maintains all mobile related information and controls each mobile handoff. The MSC also performs the functions of network management, call handling and processing, billing and fraud detection within the market.
The MSC is interconnected with the PSTN via the landline trunked lines and a tandem switch. MSCs are connected with other MSCs via dedicated signaling channels for exchange of location, validation and call signaling information. First generation wireless systems provide analog speech and inefficient low-rate data transmission between the base station and the mobile user. The speech signals are usually digitized using a standard TDM format for transmission between the base station and the MSC and are always digitized for distribution from the MSC to the PSTN.
Second-generation wireless telephone networks
- First-generation cellular telephone systems were designed primarily for a limited customer base, such as business customers and a limited number of affluent residential customers. The problems inherent with these cellular telephones were poor battery performance and channel unavailability. Improved batteries were also needed to reduce the size and cost of mobile units, especially those that were designed to be handheld. Weak signal strengths resulted in poor performance and ahigh rate of falsely initiated handoffs (false handoffs).
- It was determined that improved battery performance and higher signal quality were possible only by employing digital technologies.
- In the United States, the shortcomings of the first-generation cellular systems led to the development of several second-generation cellular telephone systems, such as narrowband AMPS (N-AMPS) and systems employing the IS-54, IS-136, and IS-95 standards.
- A second-generation standard, known as Global System for Mobile Communications (GSM), emerged in Europe. The U.S Standards of TDMA and CDMA also belong to this generation.
- Other second-generation wireless standard include, the British Cordless telephone standard CT2, Personal access Communication System (PACS) and the European standard for cordless and office telephony Digital European Cordless telephone (DECT).
- 2 G technology supports data, speech, fax, sms and WAP services.
- The architecture employed in second generation networks have reduced the computational burden on the MSC.
- GSM for example uses a base station controller (BSC) which allowed the data interface between the BSC and MSC to be standardized. This allows carriers to use different manufacturers for MSC and BSC components.
- All Second-generation systems use digital voice coding and digital modulation. The systems employ dedicated control channels within the air interface for simultaneously exchanging voice and control information between the subscriber, the base station and the MSC while the call is in progress.
- Second generation networks also provide dedicated voice and signaling trunks between MSCs and between each MSC and the PSTN.
- The first-generation systems were designed primarily for voice whereas the second-generation systems are specifically designed to provide paging, Fax and high data rate internet access.
- The network controlling structure is more distributed in second generation networks since mobile stations assume greater control functions.
- The handoff process is more mobile controlled and is known as Mobile assisted handoff (MAHO). The mobile units perform additional functions of received power reporting, adjacent base station scanning, data encoding and encryption.
- DECT (Digital European Cordless telephone) is an example of a second-generation cordless standard. It allows each cordless phone to communicate with any number of base stations. The base station with the greatest signal level is selected. The base stations have greater control in terms of switching, signaling and controlling handoffs.
- In general, second generation systems have been designed to reduce the computational and switching burden at the base station or MSC. They also provide more flexibility in the channel allocation scheme so that systems may be deployed rapidly and in a less coordinated manner.
- The limitations of 2Gare
- Low data rates ranging from 9.6 kbps to 28.8kbps
- Circuit switched network
- End systems are dedicated for the entire call duration
- Inefficient usage of bandwidth and resources
Interim 2.5 G -generation wireless telephone networks
- The need for increased throughput data rates in data transfer such as web browsing and email led to the evolution of 2.5 G which is between 2g and3G.
- The mobile technology using GPRS (General Packet Radio Service) has been termed as 2.5G.
- The 2.5 G was started in 1998 with added GPRS and enhanced data rates for GSM evolution (EDGE). In addition to the Hypertext transfer protocol (HTTP) it supports the Wireless Access Protocol (WAP) through which web pages can be viewed on the small screen of a mobile phone or a handheld device which led to the development of mobile commerce (m-commerce).
- 2.5 G is packet switched and can use some of the existing infrastructures of GSM and CDMA (Code division multiple access) networks.
Third-generation wireless telephone networks
- The aim of third generation wireless networks is to provide a single set of standards that can meet a wide range of wireless applications and provide universal access throughout the world.
- In 3 G networks the distinctions between cordless telephones and cellular telephones disappear and a universal personal communicator or personal handset provides access to a variety of voice, data and video communication services.
- 3rd generation systems use the Broadband ISDN to provide access to information networks such as the internet and other private and public databases.
- 3 G networks carry all types of information like voice, data and video.
- They operate in densely populated and sparsely populated areas.
- They serve both stationary users and vehicular users travelling at high speeds.
- Packet radio communication is used in the 3 G networks
- Personal communication System (PCS), International Mobile Telecommunication (IMT-2000) and Universal Mobile telecommunication System (UMTS) are examples of 3G wireless networks. UMTS is also known as W-CDMA (Wideband CDMA)
Fourth-generation wireless telephone networks
- 4th-generation networks emerged as a data-optimized technology with the promise of speed improvements up to 10-fold over existing 3G technologies.
- It is basically the extension in the 3G technology with more bandwidth and services offers in the 3G.
- The expectation for the 4G technology is basically the high-quality audio/video streaming over end to end Internet Protocol. The transmission rates of 4G will be up to 20Mbps higher than that of3G.
- The first two commercially available technologies billed as 4G were the WiMAX standard and the LTE standard. LTE – Advanced is the newest version of LTE.
- One of the main ways in which 4G differed technologically from 3G was in its elimination of circuit switching, instead employing an all-IP network. 4G utilizes packet switching over internet, LAN or WAN networks via VoIP.
- 4G technology is meant to provide what is known as “ultra-broadband” access for mobile devices. It is set to deliver 100 Mbps to a roaming mobile device and up to 1 Gbps to a stationary device.
- 4G will bring the perfect real-world wireless inter networking called World Wide Wireless Web.
- 4 G allows for video conferencing, streaming picture-perfect video for telemetric applications
- OFDMA multi-carrier transmission methods, frequency-domain equalization (FDE) methods, MIMO (Multiple Input Multiple Output) and Turbo Code techniques are used in 4 G networks.
- Peak data rates for 4G networks must be close to 100 megabit per second for a user on a highly mobile network and 1 gigabit per second for a user with local wireless access or a nomadic connection.
- True 4G must also be able to offer smooth hand overs across differing networks without data loss and provide high quality of service for next-gen media.
- One of the most important aspects of 4G technology is the elimination of parallel circuit-switched and packet-switched network nodes using Internet Protocol version 6 (IPv6). The currently used standard, IPv4, has a finite limitation on the number of IP addresses that can be assigned to devices
Key takeaway
Technology | Various Generations | ||||
| 1G | 2G | 2.5G | 3G | 4G |
Design began | 1970 | 1980 | 1985 | 1990 | 2000 |
Implementation | 1984 | 1991 | 1999 | 2002 | 2012- 2015 |
Service | Analogue Voice | Digital Voice | High-capacity packets, MMS | High-capacity broadband Data | High – capacity, completely IP, multimedia |
Multiple Access | FDMA | TDMA,CDMA | DMA,CDMA | CDMA | OFDMA |
Standards | AMPS, TACS,NMT | CDMA, GSM,PDC | GPRS,EDGE | WCDMA, CDMA2000 | Single Standards |
Bandwidth | 1.9kbps | 14.4 kbps | 384 kbps | 2mbps | 200mbps |
Core network | PSTN | PSTN | PSTN, Packet network | Packet network | Internet
|
The free space propagation model is used to predict received signal strength when the transmitter and receiver have a clear, unobstructed line-of-sight path between them. In free space radio signals propagate as light does i.e., they follow a straight line. If such a straight line exists between a sender and a receiver it is called line-of-sight (LOS). Even, if no matter exists between the sender and the receiver, the signal still experiences the free space loss. The received power Pr is proportional to 1/d2 with d being the distance between sender and receiver (inverse square).
Free Space Model:
- The free space model predicts that received power decays as a function of the T-R separation
- The free space power received by a receiver antenna which is separated from a radiating transmitter antenna by a distance d, is given by the Friis free space equation:
Pr(d) = PtGtGr2/(4π)2d2L
- Where, Pr(d)= Received power; Pt =Transmitted power ;Gt &Gr = Tx & Rx antenna gain respectively λ= wavelength; D =Distance; L = loss Factor
- The gain of an antenna is related to its effective aperture, by
G= 4 π Ae/ 2
- The effective aperture is related to the physical size of the antenna, and X is related to the carrier frequency by
= c/f = 2 π c/ωc
- The effective isotropic radiated power (EIRP) is defined as
EIRP = Pt.Gt
- The path loss for the free space model when antenna gains are included is given by
PL(dB) = 10logPt/Pr = -10 log[GtGr2/(4π)2d2]
- When antenna gains are excluded, the antennas are assumed to have unity gain, and path loss is given by
PL(dB) = 10logPt/Pr = -10 log[2/(4π)2d2]
This model includes not just one path, but also another path that reflects off of the ground, as shown in Figure below (in Rappaport). The model isn’t hard to develop, and provides an important theoretical underpinning to the multiple breakpoint model. Remember, powers of multipath DON’T add together. Only voltages or field strength of multipath actually add together. The voltage on the antenna is proportional to the electric field at the antenna position. So, let’s talk about adding electric fields.
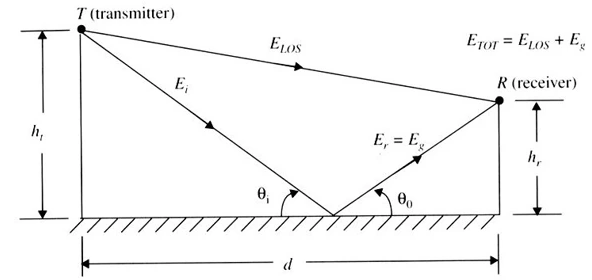
Fig 3 TX and RX are separated on the ground by d, but are at heights ht and hr respectively
Direct Path
Recall that the electric field magnitude decays as 1/d in free space. So, similar to how we wrote the received power with a reference distance d0, we write the E-field strength as the E-field strength at a reference distance, multiplied by d0/d′, for a path (distance of travel for waves) length d ′. Also, assume the signal is a simple sinusoid at the carrier frequency, fc. So,
E(d,t) = E0 ))
For the LOS path, given a distance along the ground of d, antenna heights ht and hr at the TX and RX, respectively, the
d ′ =
ELOS = E0))
Reflected Path
Two things change for the reflected path compared to the LOS path:
- The path is longer than the LOS path for total length
- The reflected field strength changes by a factor of Γ. In general, we can write the E-field as
Eg = ΓE0 ))
Let’s assume that d is very long compared to the antenna heights. So, the angle of incidence is approximately 0. In this case the reflection coefficient (assume perpendicular polarization) is -1. Then,
Eg = - E0 ))
Total Two-Ray E-Field
We are interested in the magnitude of the total E-field, ETOT = ELOS + Eg, that is, the quantity that multiplies the cos(2πfct) term. Using trig identities, and this approximation
∆ = ≈
|ETOT | ≈ 2E0 sin (
)
This means that the power decays as 1/d4! See Figure
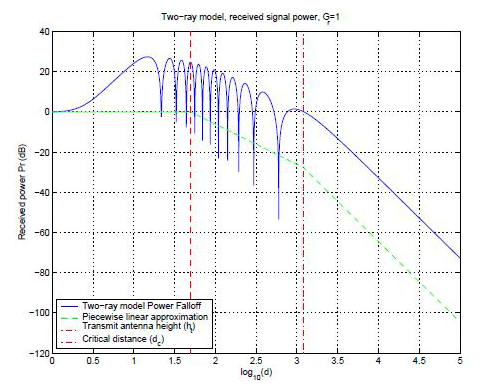
Fig 4 Received power as a function of log distance in two-ray model
Key takeaway
Remember, powers of multipath DON’T add together. Only voltages or field strength of multipath actually add together.
This model has the information which is graphical based on the Okumara model. This model is used to predict the path loss in urban areas. This mode mainly has the waves which are reflected, diffracted and scattered from the obstacles. The parameters for this model are
- Frequency Range: 150 MHz to 1500 MHz
- Transmitter Height: 30 m to 200 m
- Link distance: 1 km to 20 km
- Mobile Station (MS) height: 1 m to 10 m
For urban area the model is as below
LU = 69.55+26.16 logf – 13.28loghB- CH + (44.9-6.55loghB) logd
The area for which antenna height is >10m
CH = 0.8 + (1.1 logf – 0.7) hM – 1.56logf
For Large Cities
CH = 8.29(log(1.54hM))2 – 1.1 for 150MHz<f<200MHz
= 3.2(log(11.75hM))2 – 4.97 for 200MHz<f<1500MHz
LU= Path loss in Urban Areas
hB= Height of base station antenna in meters (m)
hM= Height of mobile station antenna in meters (m)
f= Frequency of Transmission in megahertz (MHz).
CH= Antenna height correction factor
The Hata model equation for suburban areas is given by
LSU = LU – 2(log)2 – 5.4
LSU = Path loss in suburban areas in decibels (dB)
LU = Average Path loss in urban areas in decibels (dB)
f = Frequency of Transmission in megahertz (MHz).
The Hata model for areas with less obstruction is
Lo = LU – 4.78(logf)2 + 18.33logf – 40.94
LO = Path loss in open area. Unit: (dB)
LU = Path loss in urban area. Unit: decibel (dB)
f = Frequency of transmission. Unit: (MHz)
Key takeaway
The above model has one disadvantage that it has slow response to rapid terrain changes.
Channel estimation is an important technique especially in mobile wireless network systems where the wireless channel changes over time, usually caused by transmitter and/or receiver being in motion at vehicular speed. Mobile wireless communication is adversely affected by the multipath interference resulting from reflections from surroundings, such as hills, buildings and other obstacles. In order to provide reliability and high data rates at the receiver, the system needs an accurate estimate of the time-varying channel. Furthermore, mobile wireless systems are one of the main technologies which used to provide services such as data communication, voice, and video with quality of service (QoS) for both mobile users and nomadic. The knowledge of the impulse response of mobile wireless propagation channels in the estimator is an aid in acquiring important information for testing, designing or planning wireless communication systems. Channel estimation is based on the training sequence of bits and which is unique for a certain transmitter and which is repeated in every transmitted burst. The channel estimator gives the knowledge on the channel impulse response (CIR) to the detector and it estimates separately the CIR for each burst by exploiting transmitted bits and corresponding received bits. Signal detectors must have knowledge concerning the channel impulse response (CIR) of the radio link with known transmitted sequences, which can be done by a separate channel estimator. The modulated corrupted signal from the channel has to be undergoing the channel estimation using LMS, MLSE, MMSE, RMS etc before the demodulation takes place at the receiver side. The channel estimator is shown in figure.
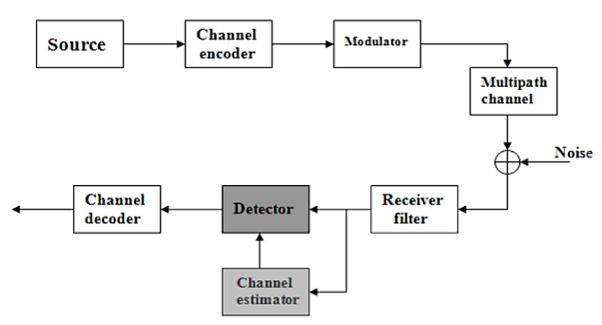
Fig 5 Block Diagram of Channel Estimator
Advanced technologies are needed to be developed in order to increase the capacity, high data rate, lower latency, and packet-optimized system that supports multiple Radio Access Technologies (RATs). The current generation of mobile telecommunication networks called the pre-4G standard, is a step towards the advanced LTE, which is an enhancement to Universal Mobile Telecommunication Systems (UMTS). LTE is a variant of the next generation of mobile telephone. The main feature of the next generation of mobile wireless communication system is to deliver high data rate. 3GPP Long Term Evolution is a project name within the third Generation Partnership Project (3GPP). The LTE project is not a standard, but it will help to modify the new UMTS mobile standard for the future requirement.
LTE is identified as the universal terrestrial radio access (E UTRA) and as the universal terrestrial radio access network (E UTRAN) which is based on conventional OFDM. The key aim of the LTE will be as good as the 3GPP High Speed Packet Access (HSPA) technology. The worldwide carriers including the ones in the United States, has announced plans to convert their current networks to LTE beginning 2009. In 2009 the European Commission also had a plan to invest about 18 million Euro in LTE deployment research and putting forward, a future 4G system. The use of DPSS together with the Fourier basis expansion is to be tested in order to model the WINNER phase II channel model. The WINNER phase II model is to be compared with the other two time-varying channel estimation methods; the Slepian basis expansion and Fourier basis expansion using basis functions K. The basis functions are to be used in order to determine the Mean Square Error (MSE) by using the Least Squares (LS) method.
The reception of a signal in a channel transmitted through any type of fading channel degrades in quality if the signal level attenuation is below the expected operation region of the receiver. In this situation, the received signal power is not expectedly enough comparing with signal noise and interference power for reliable reception. The solution to overcome the channel attenuation because of fading problem in channel is to increase the transmitted power adjusted to the attenuation which is called power control (PC). On the other hand, there are two primary problems with this power control (PC) system.
One of these problems is that the dynamic range of the transmitter and the required transmitting power is extremely high if it’s intended to fully compensate the fading. This is impossible because of the radiation power limitations, the cost and the size of the amplifiers, and the limited battery power in the portable unit. Moreover, excess transmitted power increases the interference level at the other channels and users in the system unit. Another problem in power control (PC) approach that a feedback link is needed for the channel unless the operation of the radio channel is in time division duplex (TDD) mode. In a TDD system, the same frequency band is used for the downlink transmission from the base station to mobile unit and for the uplink transmission from the mobile unit to the base station. As a result, the transmitted signal undergoes the fading channel as the received signal due to its reciprocal characteristic of the channel, the transmitted power of transmitter is adjusted according to the received signal power. The feedback information usage decreases throughout the channel and increases the complexity in the system. Even an appropriate feedback link may not available in some application.
Using PC the fading can’t be overcome completely but the attenuation may compensate considerably. It can mention that large-scale fading can be compensated as well in the uplink of a system, for example CDMA. But stringent power control is required in prevention near far problem in the system. The rate of large-scale fading is simply slow, as a result it can be tracked well and the delay in the feedback of the power control commands can be neglected comparing with the rapid fading. On the other hand, small-scale fading can result in such rapid variations in the signal power that even the power control can’t follow them.
Diversity Techniques:
Diversity technique is used to decreased the fading effect and improve system performance in fading channels. In this method, we obtain L copies of desired signal through M different channels instead of transmitting and receiving the desired signal through one channel. The main idea here is that some the signal may undergo fading channel but some other signal may not. While some signal might undergo deep fade, we may still be able to obtain enough energy to make right decision on the transmitted symbol from other signals. There is a number of different diversities, which is commonly employed in wireless communication systems. Some of them are following:
- Multipath/frequency diversity
- Spatial/space diversity
- Temporal/time diversity
- Polarization diversity
- Angle diversity
- Antenna diversity
Multipath or Frequency Diversity:
In a channel, Transmitted signals with different frequencies are affected different way in frequency domain. The fact is an advantage in frequency diversity technique. Multiple replicas of information signal are sent over several affected frequency band in this diversity. There should be a distance more than coherent bandwidth between the frequency bands and achieve small-scale fading according to following equation.
fc = 1/fd
Frequency diversity can also be implied as in the case of multipath diversity. Transmission of a wideband signal is given by the following equation where the bandwidth is more than the coherence bandwidth of the previously used channel and this results a frequency selective fading
fc = 1/tm
In a sufficient wide signal bandwidth, multipath components can resolve. In the result, it is possible to obtain different independently fading signal. The number of resolvable multipath given by the following equation is used to approximate the maximum achievable diversity order for multipath diversity:
L= [Tm + W] +1
Channel equalization is another approach to achieve multipath diversity. A filter is used at receiver to make channel equalization to compensate the channel impairments. This process combines the multipath of signals and reduces inter-symbol-interference (ISI) and produce diversity.
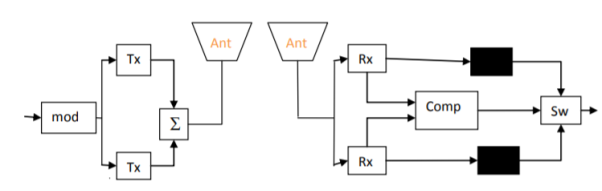
Fig 6 Frequency Diversity
In general, the information signals are modulated through different carriers M in frequency diversity scheme. It is important that different signals undergo independent fading. The carriers should be separated by at least coherent bandwidth from each other. L copies of signals are optimally combined at the receiver to make a statistic decision. The maximum ratio combiner is the optimal combiner.
Spatial/Space Diversity:
Multiple antennas are used to transmit signals with carrying information at the transmitter and/or receiver to provide multiple independent fading paths in space diversity. This technique is used to provide significant performance gain with not sacrificing any valuable bandwidth on the transmitted power resources. Spatial diversity is widely used because it is easy to implement and it’s cost effective and very simple. This technique has a single transmitting but multiple receiving antennas. The receiving antennas should be at enough distance for that the multiple fading in the diversity will be uncorrelated. There should be a balanced average power between channels and the correlation coefficient should be very low to achieve a good diversity gain.
While wide distance is required between antennas for obtaining low correlation between channels but close distance is also required to synthesize to make a narrow beam not generating grating lobes which prevent introducing interference. Only one or two co-channel interfering signals are used in time division multiple access (TDMA) systems. However, the number of signals is more in code division multiple access (CDMA) the maximal ratio combining (MRC) is better than the performance of optimum adapting processing. It is suggested that if we increase antenna element separation as much as feasible the then high space diversity gain can be achieved with maximal ratio combining in a CDMA system. Most of the cellular communication system has only one transmitting antenna at the base station and two receiving antennas those are widely separated per sector in a space diversity system. In general, if we want to receive M copies of transmitted signals then we need M number of antennas in a space diversity system.
It is very important to keep enough space between the antennas so that the received signals undergo independent fading. Space diversity is different than frequency and temporal diversity. Unlike those spaces diversity needs no additional work at the transmission end and no additional bandwidth is required on the transmission time.
On the other hand, physical complexity restricts its application widely. Like several receiving antennas use in space diversity, several transmission antennas also can be used to send several copies of transmitted signals. This kind of diversity can be employed combating frequency and time selective fading both. There are two types of spatial diversity techniques such as receive diversity and transmit diversity.
Receive Diversity: Multiple antennas are use at the receiver to obtain diversity and employ switching and combining or selection intending to improve the quality of received signal. Since it is easier and cost effective to use multiple antennas at the base station than the terminal which is a positive manner of receive diversity. This technique may utilize channel state information (CSI) at receiver and it’s fully fit for uplink which is remote to base. But the main problems of receive diversity are cost, size and necessary power at the remote units. This technique is larger in size and expensive in cost because of multiple antennas, radio frequency chains or selections and its switching circuits.
Transmit Diversity: Unlike receive diversity, transmit diversity needs multiple transmitting antennas. Moreover, unlike receive diversity, transmit diversity does not utilize CSI in its single information signal. Effective signal processing technique should be used to extract the noisy and distorted received signal in transmit diversity.
Time / Temporal Diversity: Interleaving and coding, over symbols across different coherent time periods, is used to obtain time or temporal diversity. This technique utilizes coding of channel and interleaving to mitigate channel fading at a cost of added delay and loss of bandwidth efficiency. It is uses on slow fading channels and on the channels which is delay sensitive. Intentional redundancy is introduced into the transmitted signal to achieve time diversity in the temporal domain. Redundancy can be done by repetition of channel coding. To make repetition coding, information bearing signals are transmitted in several time slots. But the separation between time slots should be more or equal than the coherent time of the channel to obtain independent faded signals which helps to gain full diversity advantages. Moreover, it is possible to obtain repetition coding by spreading in direct-sequence code division multiple access (DS-CDMA).
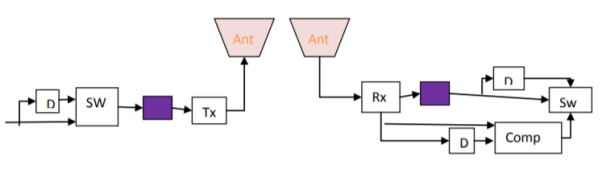
Fig 7 Time Diversity
In general, a desired signal is transmitted in M different periods of time in time diversity. For example, every symbol is transmitted M times. As it is mentioned earlier that intervals between the transmitted symbols should be at least coherence time to make ensure that different copies of the same symbol undergo independent fading. Maximum ratio combiner can be used to obtain optimal combiner. If we send the same symbol M times then it applies the (M, I) repetition code. We can also use non-trivial coding. Error control coding and interleaving is an effective way to combat time selective or fast fading.
Polarization Diversity: In polarization diversity, transmitted signals have uncorrelated fading statistics in VHF and VHF land mobile radio system when signals should be transmitted through two orthogonally propagations path. Spatial diversity may achieve by using multiple antennas with independent polarizations in the same location instead of multiple antennas in use in different locations. This is the method of polarization diversity. If an implementation of spatial diversity with small dimensions is desired, this is very attractive process. Normally two orthogonally polarized antennas are used on horizontal and vertical planes or with a slope of 150 to employ polarization diversity. Experiments show that polarization diversity may obtain in dense scattering environments when there is line of sight (LOS) and non-line of sight (non-LOS) situations.
Angle Diversity/ Pattern Diversity / Direction Diversity:
Equal data traffic is used on the both uplink (reverse link) and downlink (forward link) in digital cellular communication but the system requires better reverse link performance because of the limitation of mobile terminal transmit power. There is uplink capacity deployed in CDMA system due to synchronize operation on forward link and asynchronies operation on reverse link. If we need to achieve better uplink reliability then we can use space diversity or polarization diversity. On the other hand, there is a huge demand of data applications on downlink capacity comparing to the uplink capacity. Improvement of reliability or downlink capacity is a major issue in three generation mobile system and the other generation mobile communication systems in future. One promising solution to improve downlink capacity is forming beam on downlink. It can also be implemented by forming multiple fixed narrow beams or by steering a beam toward a user in the system.
Additional protection which the angle diversity provides is protection from deep multipath fading in multiple beam systems. It is experimentally proved that the angle diversity is equally effective as conventional space diversity in dense urban area and it provides approximately 8 dB diversity gain at reliability level with selection combining. A multiple fixed narrow-beam antenna or an array antenna, which is fully adaptive, transmits and receives much more energy between the mobile terminal and base station comparing to a wide beam antenna system. On the other hand, a beam forming system using multiple antennas may experiences multipath fading sometimes when the multipath components are applied from very close angles.
The base station antenna system in a dense urban environment experiences antenna gain reduction and the multipath components will spread in wider angles. In this case, it is wise to use angle diversity to avoid deep multipath fading by choosing the best beam to collect energy. The size of the angle diversity is very smaller comparing to the space diversity system where we need the wide separation between the receiving antennas.
Multipath components in a cluster those have individual arrival angles travel through different paths and employ different fading. Very basic procedure to obtain angle diversity is to fix antennas with narrow beam widths different sector in the system. Then the arriving multipaths from the different beam directions are resolved and combined advantageously. This procedure not only creates diversity but also increases the antenna gain and reduces interference by providing angular discrimination. It is mentioned earlier that one more method of achieving diversity is to use antenna array with adaptive beam forming which termed as path diversity sometimes.
Antenna Diversity:
Antenna diversity is a popular and extensively used technique to improve performance in wireless communication systems. The technique reduces fast fading and inter-channel interference effects in the wireless network system. In an antenna diversity system, two or more antennas are used and fixed in positions which will provide uncorrelated signals with the same power level. Then the signals are combined and created an improved signal. The basic method of antenna diversity is that the antennas experience different kind of signals because of individual channel conditions and the signals are correlated partially. Then we can expect that if one signal from one antenna is highly faded, other signals from other antennas are not faded such way and these signals are our expected quality signals. In a multipath propagation environment, each receiving signal experiences individual fading characteristic.
Key takeaway
- Using PC the fading can’t be overcome completely but the attenuation may compensate considerably.
- It can mention that large-scale fading can be compensated as well in the uplink of a system, for example CDMA. But stringent power control is required in prevention near far problem in the system.
- The rate of large-scale fading is simply slow, as a result it can be tracked well and the delay in the feedback of the power control commands can be neglected comparing with the rapid fading.
- On the other hand, small-scale fading can result in such rapid variations in the signal power that even the power control can’t follow them.
References:
1. Cristopher Cox, “An Introduction to LTE: LTE, LTE-Advanced, SAE, VoLTE and 4G Mobile Communications”, Wiley, 2nd Edition.
2. E. Dahlman, J. Skold, and S. Parkvall, “4G, LTE-Advanced Pro and The Road to 5G”, Academic Press, 3rd Edition.
3. B. P. Lathi, “Modern Digital and Analog Communications Systems”. Oxford university press, 2015, 4th Edition.
4. Obaidat, P. Nicopolitids, “Modeling and simulation of computer networks and systems: Methodologies and applications” Elsevier, st Edition.