Unit - 1
Evolution of Structure of Power Systems
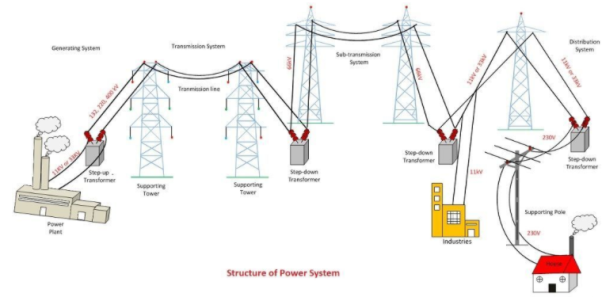
Figure. Power System Structure
Generating Substation
In generating station the fuel like coal, water, nuclear energy, etc. is converted into electrical energy. The electrical power is generated in the range of 11kV to 25kV, which is step-up for long distance transmission. The power plant of the generating substation is mainly classified into three types, that is thermal power plant, hydropower plant and nuclear power plant.
The generator and the transformer are the main components of the generating station.
Transmission Substation
The transmission substation carries the overhead lines which transfer the generated electrical energy from generation to the distribution substations. It only supplies the large bulk of power to bulk power substations or big consumers.
The transmission lines mainly perform the two functions
- It transports the energy from generating stations to bulk receiving stations.
- It interconnects the two or more generating stations. The neighbouring substations are also interconnected through the transmission lines.
Sub-transmission Substation
The portion of the transmission system that connects the high voltage substations through the step-down transformer to the distribution substations is called the sub-transmission system.
The sub-transmission voltage level ranges from 90 to 138KV. The sub-transmission system directly serves some large industries.
Distribution Substation
The component of an electrical power system connecting all the consumers in an area to the bulk power sources is called a distribution system. The bulk power stations are connected to the generating substations by transmission lines. They feed some substations which are usually situated at convenient points near the load centres.
Key Takeaways:
An electric power system is usually understood as a very large network that links power plants (large or small) to loads, by means of an electric grid that may span a whole continent
The power gadget is the complex agency that can be subdivided into the subsequent sub-structures. The subsystems of the power device are explained underneath in details.
Generating substation:
In producing station the gasoline (coal, water, nuclear electricity, and many others.) is transformed into electric electricity. The electrical energy is generated in the variety of 11kv to 25kv, that is step-up for long distance transmission. The power plant of the producing substation is especially classified into three types, i.e., thermal power plant, hydropower plant and nuclear energy plant.
The generator and the transformer are the main components of the producing station. The generator converts the mechanical energy into electrical power. The mechanical electricity comes from the burning of coal, gasoline and nuclear gasoline, gas mills, or sometimes the inner combustion engine.
The transformer transfers the energy with very excessive performance from one level to every other. The energy switch from the secondary is about same to the primary besides for losses within the transformer. The step-up transformer will lessen losses in the line which makes the transmission of electricity over long distances.
Transmission substation:
The transmission substation incorporates the overhead traces which switch the generated electric energy from technology to the distribution substations. It only materials the big bulk of energy to bulk energy substations or very massive customers.
The transmission strains especially perform the 2 functions
- It transports the energy from generating stations to bulk receiving stations.
- It interconnects the 2 or extra producing stations. The neighbouring substations also are interconnected thru the transmission lines.
The transmission voltage is working at greater than 66kv and is standardised at 69kv, 115kv, 138kv, 161kv, 230kv, 345kv, 500kv, and 765kv, line-to-line. The transmission line above 230kv is normally called greater excessive voltage (ehv).
The high voltage line is terminated in substations which can be known as excessive voltage substations, receiving substations or primary substations. In excessive voltage substation, the voltage is step-all the way down to a appropriate cost for the following a part of drift closer to the load. The very huge business customers can be served directly to the transmission system.
Sub-transmission substation:
The portion of the transmission system that connects the excessive voltage substations via the step-down transformer to the distribution substations is referred to as the sub-transmission system.
The sub-transmission voltage degree degrees from 90 to 138kv. The sub-transmission system at once serves a few massive industries. The capacitor and reactor are placed inside the substations for keeping the transmission line voltage.
The operation of the sub-transmission system is similar to that of a distribution system. Its differ from a distribution machine in the following way.
A sub-transmission machine has a higher voltage level than a distribution gadget.
It resources best bigger hundreds.
It resources only some substations compared to a distribution machine which components a few loads.
Distribution substation:
The issue of an electrical power system connecting all of the consumers in an area to the majority energy sources is referred to as a distribution machine.The majority electricity stations are related to the producing substations via transmission strains. They feed a few substations which are typically located at handy points near the load centres.
The substations distribute the energy to the home, business and comparatively small clients. The consumers require huge blocks of energy which might be normally supplied at sub-transmission or maybe transmission gadget.
Electric technology became based at the awesome discovery by faraday that a converting magnetic flux creates an electric powered Field. Out of that discovery, grew the largest and maximum complicated engineering success of guy: the electrical energy device.
Certainly, life without power is now impossible. Electric power systems shape the fundamental infrastructure of a rustic. Even as We examine this, electrical energy is being produced at charges in extra of hundreds of giga-watts (1 gw = one million,000 w).
Large rotors spinning at hastens to 3000 rotations in keeping with minute convey us the energy stored inside the potential energy of water, or In fossil fuels. Yet we note power only whilst the lights go out!
At the same time as the fundamental capabilities of the electrical power machine have remained nearly unchanged inside the beyond century, there are Some good sized milestones inside the evolution of electrical power structures:
First entire dc power machine built by using edison (1882): incandescent lamps supplied by means of steam driven dc turbines (electrical cable machine at 110v). 59 clients unfold over an approximate vicinity with 1.5 km radius.
Improvement of transformers brought about supersession of dc systems by means of ac structures.
Nikola tesla - polyphase induction cars: led to improvement of ac three segment systems.
Interconnection of systems caused standardization of frequency (why?), 60 hz in north the us and 50 hz in most Other international locations. Use of higher and better voltage tiers (up to a thousand kv line-line rms ac). Standardization of voltage Levels.
Development of mercury arc valves, and sooner or later thyristors caused excessive voltage dc transmission (hvdc): dc Transmission appropriate for terribly lengthy distance bulk transmission and underwater cable hyperlinks. First commercial dc link in 1954.
Numerous new trends: gasoline mills, static excitation structures, speedy acting circuit breakers, microprocessor based totally Relaying, use of communique technologies etc.
Want for better utilisation and operation of ac transmission systems by way of use of high electricity electronic converters Advised. Several such converters at the moment are in operation.
Smart Grid
A Smart Grid is an electricity Network based on Digital Technology that is used to supply electricity to consumers via Two-Way Digital Communication. This system allows for monitoring, analysis, control and communication within the supply chain to help improve efficiency, reduce the energy consumption and cost and maximise the transparency and reliability of the energy supply chain.
The areas of application of smart grids include: smart meters integration, demand management, smart integration of generated energy, administration of storage and renewable resources, using systems that continuously provide and use data from an energy network.
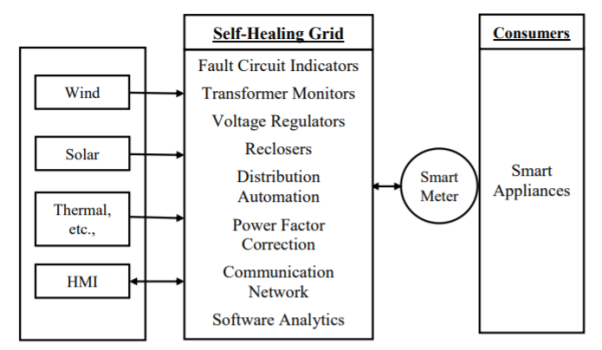
A smart grid automatically detects and responds to routine problems and quickly recovers if they occur, minimising downtime and financial loss. The Self-Healing Grid is a system comprised of sensors, automated controls, and advanced software that utilizes real-time distribution data to detect and isolate faults and to reconfigure the distribution network to minimize the customers impacted. One of the main goals of a Self-Healing Grid is to improve system reliability. This can be accomplished by reconfiguring the switches and reclosers installed on the distribution feeder to quickly isolate the faulted section of the feeder and re-establish service to as many customers as possible from alternate sources/feeders.
A Smart Grid is an electricity network that can intelligently integrate the actions of all users connected to it – generators, consumers and those that do both – in order to efficiently deliver sustainable, economic and secure electricity supplies.
- System (Generation, Transmission, Distribution) with an advanced twoway communications system
- Enables real-time monitoring and control
- Provide greater visibility and transparency
- Consequently, enables cost reduction and efficiency improvement Smart Grid is based on Digital Technology that is used to supply electricity to consumers via Two-Way Digital Communication.
This system allows for monitoring, analysis, control and communication within the supply chain to help improve efficiency, reduce the energy consumption and cost and maximise the transparency and reliability of the energy supply chain. The flow of electricity from utility to consumer becomes a two-way conversation, saving consumers money, energy, delivering more transparency in terms of end-user use, and reducing carbon emissions.
Functions of Smart Grid Components
The areas of application of smart grids include: smart meters integration, demand management, smart integration of generated energy, administration of storage and renewable resources, using systems that continuously provide and use data from an energy network
Smart Devices Interface Component
Smart devices for monitoring and control form part of the generation components real-time information processes. These resources need to be seamlessly integrated in the operation of both centrally distributed and district energy systems.
Storage Component
Due to the variability of renewable energy and the disjoint between peak availability and peak consumption, it is important to find ways to store the generated energy for later use. Options for energy storage technologies include pumped hydro, advance batteries, flow batteries, compressed air, super-conducting magnetic energy storage, super capacitors, and flywheels.
Transmission Subsystem Component
The transmission system that interconnects all major substation and load centers is the backbone of an integrated power system. Transmission lines must tolerate dynamic changes in load and contingency without service disruptions. Efficiency and reliability at an affordable cost continues to be the ultimate aims of transmission planners and operators.
Monitoring and Control Technology Component
Intelligent transmission systems/assets include a smart intelligent network, self monitoring and self-healing, and the adaptability and predictability of generation and demand robust enough to handle congestion, instability, and reliability issues. This new resilient grid has to withstand and be reliable to provide real - time changes in its use.
Intelligent Grid Distribution Subsystem Component
The distribution system is the final stage in the transmission of power to end users. Primary feeders at this voltage level supply small industrial customers and secondary distribution feeders supply residential and commercial customers. At the distribution level, intelligent support schemes will have monitoring capabilities for automation using smart meters, communication links between consumers and utility control, energy management components, and AMI.
Demand Side Management Component
DSM options provide reduced emissions in fuel production, lower costs, and contribute to reliability of generation. These options have an overall impact on the utility load curve. Demand side management options and energy efficiency options developed for effective means of modifying the consumer demand to cut operating expenses from expensive generators and defer capacity addition.
Microgrid
A microgrid is a localized group of interconnected loads1 and distributed energy resources (DERs) within clearly defined electrical boundaries (e.g., cities, communities, campuses that act as a single controllable entity with respect to the main regional or national electric grid. A microgrid can connect or disconnect from the main grid as physical and/or economic conditions dictate. It can operate on both grid-connected mode (synchronous with the grid), or on island mode (functioning autonomously and disconnected from the main electric grid). A typical microgrid layout is shown in Figure.
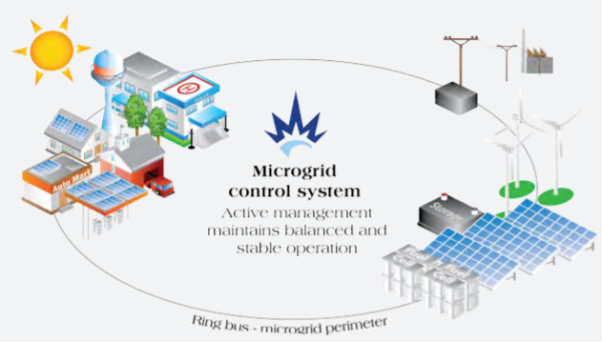
Microgrids, in a more specific sense of the term, refer to grid-connected setups of varying scale that can be “islanded” at will, and established primarily for local reliability, resilience, and operational economics. Islanding is a condition in which distributed generators (DG) continue to provide power in a location even without the continued presence of electrical grid power. This handbook focuses on these island able microgrids.
The most common objective of a microgrid, especially in the commercial and industrial (C&I) and mission-critical sectors, is to be more reliable and resilient than the utility so that the energy end user continues to have uninterrupted service even during extreme circumstances (e.g., earthquakes, storms, or power grid outages from faults on the utility grid). These “high 9” applications (referring to the level of availability ranging from 99.9% to 99.999%) are usually in hospitals, data centers, military bases, laboratories, etc. In these applications that require a high 9 level of reliability, the microgrid’s cost is viewed as secondary to the purpose, i.e., maintaining energy supply to the facility even under extreme conditions. In situations like these, the value of electricity is often several multiples of its actual cost.
Components of Microgrid
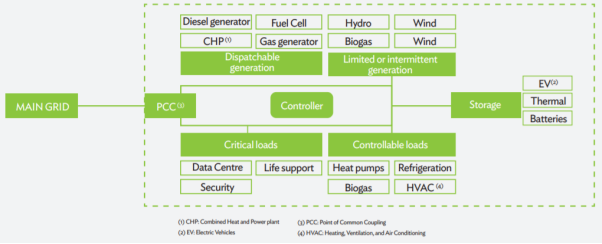
Local Generation
A microgrid must be able to supply energy to its connected loads independent of the utility, so generation sources must exist within the microgrid. These sources could range from readily controlled to intermittent, to not controllable. This could be renewable (e.g., solar PV, wind, biomass; or less frequently used such as solar thermal, hydro, micro-hydro, tidal) or nonrenewable (e.g., diesel, NG, combustion turbines, reciprocating engines, cogeneration, CHP, turbines, microturbines). The selection of energy source needs to be adjusted to the demands on the microgrid, such as the desired generating capacity, required firmness level,8 ramp rate, renewable energy targets, and availability of fuel (and fuel storage requirements). Brownfield microgrid setups often start with some form of existing generation already onsite, such as existing diesel generators, solar panels, or cogeneration facilities.
The existing generation resources influence the additional distributed generation capacity required to be added for supporting the microgrid. Recent decreases in the price solar PV modules and other forms of renewable energy such as wind and biomass, along with energy storage, have enhanced the economic feasibility and attractiveness of renewable energy-based microgrids.
End-Use Loads and Demand-Side
Energy Management The type of end-use devices determines the electrical loads that the microgrid has to be capable of handling, which in turn influences the power-generating capacity and storage requirements. For instance, cell phone charging imposes minimal capacity requirement on the system, but connecting an air conditioner to a solar PV-based microgrid can cause challenges in managing electric loads because of its large continuous power consumption. In reality, a microgrid does not consist solely of one of these extremes, but will have a range of end uses to be served.
Microgrid energy loads range in controllability characteristics from critical loads like data centers or hospital life support equipment at one end of the spectrum, to adjustable controllable loads like air conditioning, lighting, or grid dispatch at the other end. This latter category of loads can be temporarily curtailed or adjusted in line with flexible user requirements, and most modern microgrids will have the capability to control these end uses to optimize the utilization of generation and storage resources.
Demand-response (DR) is defined by Federal Energy Regulatory Commission as “changes in electric usage by end-use customers from their normal consumption patterns in response to changes in the price of electricity over time, or incentive payments designed to induce lower electricity use at times of high wholesale market prices or when system reliability is jeopardized.” DR is a subset of DSM, which includes immediate real-time measures as well as long-term and permanent energy efficiency measures. OpenADR is a standard that supports a range of automated demand-response signals from the utility’s demand-response automation server (DRAS) to automatically control connected equipment in the end-user premises.
Storage
Microgrids must have some form energy storage as a means to time-shift own generation to match load demands, as well as a critical fallback supply in islanded mode operation. With energy storage, the microgrid can absorb and store the energy generated at times when supply exceeds demand, and to subsequently return it at times when demand exceeds supply (e.g., evening peaks once solar PV generation is not available). While there are some inefficiencies from storage and line losses, these are minimal compared to the value that storage can deliver. Energy storage can also be leveraged for arbitrage in wholesale power markets or with time-based rates such as real-time, peak, and ToU pricing. The energy storage technology is chosen based on the size of the microgrid and reaction time required. For instance, for remote microgrids or smaller grid-connected microgrids, batteries are the most commonly used storage technology because the storage capacity required by the microgrid does not justify the higher costs associated with other storage technologies. Batteries can also be used to provide the ancillary services required within a microgrid. Large-scale storage technologies, such hydro-based storage or thermal storage, while cheaper for time shifting operations, have high initial costs that make their implementation in smaller and microgrids challenging.
Microgrid Monitoring and Control System
Microgrid monitoring and control systems (MMCS) tie all of the microgrid components together and maintain the real-time balance of generation and load. This control system can follow a centralized or decentralized scheme. In the most basic of microgrid configurations, the control system might just be a governor controlling a diesel generator. In more complex microgrids, the MMCS comprises sensors, metering, sophisticated software platforms, and communication paths designed to allow for real-time optimization and control of the generators, energy storage, loads, and utility interface. During grid-connected operation, the MMCS needs to manage the utility interconnection and communicate with the system operations center of the utility (or independent system operator), including any DR management systems in near real time.
Utility Interconnection
A distinctive feature of microgrids is the interconnection with the utility’s power grid, sometimes referred to as the PCC. This is what allows microgrids to operate in grid-connected or islanded modes, and transition is relatively seamlessly between both. If there is an existing utility interconnection, it lays the foundation for what additional hardware may be needed for the microgrid. This includes elements ranging from existing relays and meters in the substation and distribution equipment, to switchgears and power converters in the microgrid.
During grid-connected operation, the microgrid-utility interconnection at the PCC must be designed to allow for safe and reliable parallel and concurrent operation of the microgrid and the utility macrogrid. The existing utility equipment may need to be upgraded to handle the various changes that occur when islanding a microgrid. For reliability and resilience, focused microgrids where relatively frequent islanded mode operation is anticipated; the PCC must also incorporate equipment to allow for seamless disconnection and reconnection of the microgrid to the power grid. This re-synchronization of the two systems is a complex process and failure to adequately design for this function can result in instability of both the microgrid and the main power grid. Accordingly, islanding of microgrids must be addressed at both technical and policy levels (safety concerns, potential end user equipment damage, reclosing scenarios, inverter prioritization).
Key takeaway
A smart grid distribution system, whose objective is to develop a power grid more efficient and reliable, improving safety and quality of supply in accordance with the requirements of the digital age.
- Higher Penetration of renewable resources or distributed generation
- Extensive and effective communication overlay from generation to consumers
- Use of advanced sensors and high speed control
- Higher operating efficiency.
- Greater resiliency against attacks and natural disasters
- Automated metering and rapid power restoration
- Provided greater customer participation
Comparison of conventional grid and smart grid
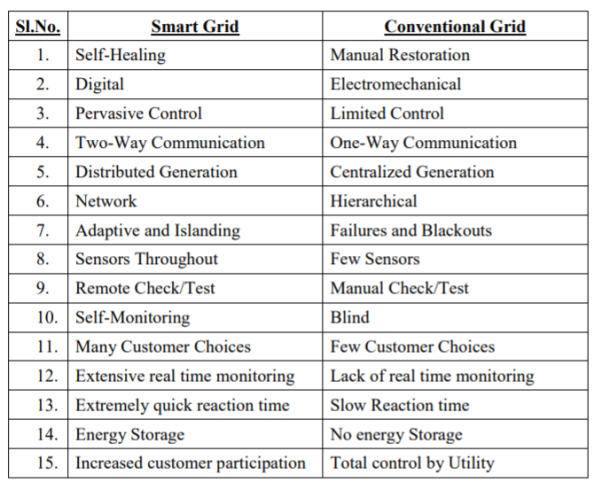
The following voltage levels are recognised in India as per I5-2026 for line to line voltages of 132 KV and higher.
Nominal system Voltage kV 132 220 275 345 400 500 750
Maximum operating Voltage kV 145 245 300 362 420 525 765
Overhead line | Underground Cable |
1) Open to sunlight, storm moist atmosphere, rain, etc.
2) Looks crowded network & spoils the beauty of good localities
3) Cheap in cost
4) Fault detection is easy
5) Maintenance is frequent
6)In case of a fault, joining /replacing is easy
7) Faults detected & repaired in less time & consumers effected for a short time
8) The time required for erections more
9) The line is affected by facing trees
10) In populated areas, there is much more rick & chance of an accident
11) disturbance to telecommunication
| Burned underground and therefore not affected & exposed
Network not seen & beauty of locality not spoiled
Very costly
Fault detection requires a special test
Maintenance is occasional
In case of a fault, joining is tedious & time consuming
Repairs take a lot of time and consumers
Cable laying takes less time
No such chance
Risk is rare
No such chance as the lead sheath is provided |
The following points have been assumed for the overhead system:
- Same power (P watts) transmitted by each system.
- The distance (l metre) over which power is transmitted remains the same.
- The line losses (W watts) are the same in each case.
- The maximum voltage between any conductor and earth (Vm) is the same in each case.
1) DC System:
a) Dc 2 – Wire System with midpoint earthed:
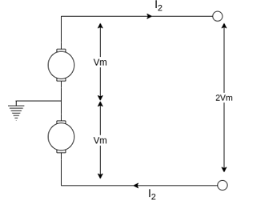
The max Voltage between any conductor & earth is “V”
Maximum voltage between conductors = 2Vm Volts
Load Current I2 =
Let a be the area of cross-section of the conductor.
The volume of conductor material required = 2al
Line Losses, W = 2 (I2)2R2 = 2 [
]
W =
Area of cross-section, a =
Volume of conductor required = 2al = 2 =
Let this volume be noted by K
2) 1- 2-write system with mid Point earthed
Assuming the power factor of the load to be cos Φ,
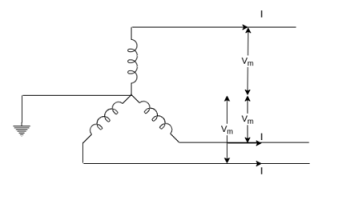
The peak value of Voltage between conductors = 2 Vm Volts
Rms value of Voltage between conductors = =
Vm Volts
Load current, I =
Line Losses W = 2I2R
= 2
=
a =
Volume of conductor material required = 2al
= 2 =
=
3) 3-Ac system
a) 3- 3-wire system:
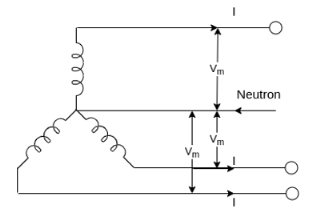
Voltage across each phase = Vm
RMS value of voltage per phase = Vm/
Power transmitted per phase = P/3
Load current per phase, I = =
Line losses W = 3I2R
= =
=
The volume of conductor material required:
= 3 a l
=
=
b) 3- 4-wire system:
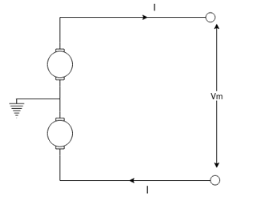
Assuming balanced load, there will be no current in neutral wire and copper loss be the same as in 3- 3 wire system
i.e. W =
a =
Taking cross-section of neutral wire as half of the line conductor
The volume of conductor material required
=3.5al
=
= K
II) The volume of conductor required for underground systems the assumptions are:
(i) In all cases, the power to be transmitted is the same (‘p’ watts)
(ii) The distance over which the power is to be transmitted is the same (l)
(iii) The line losses are the same (w watts)
(iv) The max voltage between two conductors is the same (Vim volts)
1) Dc- 2 wire system with midpoint earthed
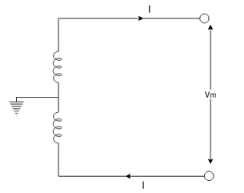
Voltage between two conductors = Vm volts
The load current, I =
Line losses W = 2I2R
=
=
Volume of conductor material required = 2al
= 4 = K
2) 1- 2 – wire system with midpoint earthed:
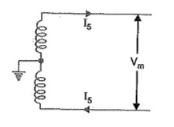
Max Value of voltage between outer conductors = Vm volts
RMS Value of Voltage between outer conductors = Vm/watts
The load current, I = =
Line losses, W = 2I2 R = 2
W =
a =
Volume of conductor material required
= 2a l
=
=
3) 3- A C System:
a) 3- 3- wire system:
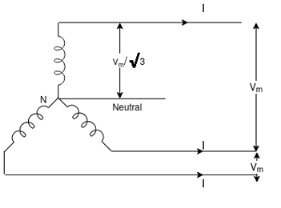
Max value voltage between conductors = Vm
RMS Value of voltage between conductors = Vm/
R.M.S Value of voltage per phase = =
The load current, I = =
Line losses W = 3I2R =
W =
=
The volume of conductor material required = 3al
=
=
b) 3- 4 –wire system:
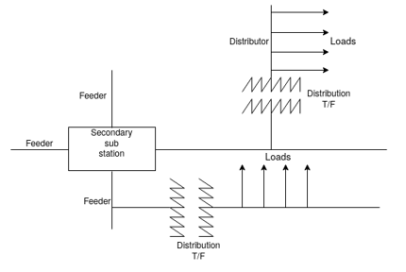
If the loads are balanced, then particular wire carries do current consequently, the system reduces to 3-, 3- wire system except that there is the additional neutral wire. Assuming the area of cross-section of the neutral wire to be half that of the conductor. So, the volume of conductor material required
= 3.5 al
=
=
HVAC | HVDC |
It stands for High Voltage Alternating Current. | It stands for High Voltage Direct Current. |
It uses a transformer for voltage conversion. | It uses solid-state semiconductor based converters for voltage conversion. |
The HVAC voltage conversion is simple & inexpensive. | The HVDC voltage conversion is complex & expensive. |
HVAC transmission requires a minimum of three conductors. | The HVDC requires only two conductors in bipolar & one conductor in monopolar transmission. |
The HVAC transmission is used for less than 600 Km distance. | The HVDC transmission is used for transmitting bulk power more than 600Km distance. |
It offers better flexibility allowing multiple tappings in the pathway. | It does not offer flexibility & transmit power from point to point. |
The effective power transmission capability is affected by reactive power losses. | There are no reactive power losses in HVDC transmission. |
The conductors are affected by corona losses. | The corona losses in HVDC are reduced by a factor of approximately 3. |
It requires a large diameter cable due to skin effect. | The current stays uniform in cable thus it can use comparatively smaller diameter cable. |
It wastes almost 30% of conductor capacity due to AC peak ratings while delivering average power. | It utilizes full conductor capacity due to the DC peak & average being the same value. |
The HVAC has a broader right-of-way. | The HVDC has a narrower right-of-way. |
The HVAC transmission requires tall sized towers. | The HVDC transmission requires a smaller tower. |
It is not preferred for submarine or underwater power transmission due to the stray capacitance of the cables. | The HVDC is preferred & used for submarine power transmission offshore. |
HVAC does not offer controllability. | It offers great controllability over the power flow, frequency control & dampening of power swings. |
The AC generates interference in the nearby communication lines. | The direct current does not generate any interference. |
The line arc may extinguish because of the voltage zero-crossing. | The arc will never get a chance to extinguish unless the power source is tripped. |
The HVAC circuit breakers are simple & cheaper because the arc will self-extinguish. | The HVDC circuit breakers are expensive & have complex design circuit to force the current to zero. |
The HVAC is best suitable for overhead power transmission at distances below 600 km which is economical. | The HVDC is used for long-distance bulk power transmission including underwater as well as the asynchronous interconnection between AC grids. |
Multiterminal HVDC system
There are 3 specific areas of applications for MTDC systems.
1. Bulk power transmission from several remote generating stations to several load centres.
Here a system of two generating stations and two loads is shown below.
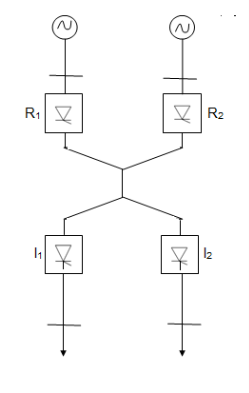
This is a radial system with two rectifiers and two inverters.
For ensuring the same level of flexibility in energy exchange, three two terminal DC links will be required in addition to a link connecting the two receiving systems which could be AC or DC.
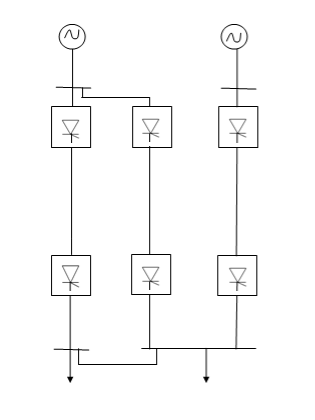
This could result in extra costs for the convertor stations, lines and additional power losses in increased number of conversions.
2. Asynchronous interconnection between adjacent power systems.
3. Reinforcing of an AC network which is heavily loaded.
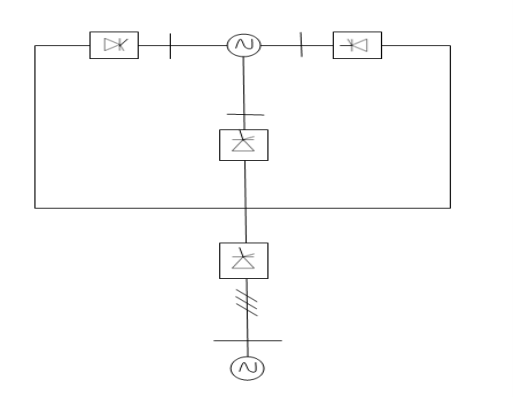
Consider an urban power system which is fed by a distant power station. It would be advantageous to arrrange the power injection at more than one point so that the underlying AC network is not overloaded.
Types of MTDC systems-
1)
Series MTDC system- This is a natural extension of the two terminal system which is a series connected system. A three terminal MTDC system with a monopolar arrangement is shown as:
In one converter station would require either the disconnection of a bridge in all the stations or disconnection of the affected station.
Comparison of series and parallel MTDC system
(i) High speed reversal of power is possible in series systems without mechanical switching. This is not possible in parallel systems.
(ii) The valve voltage rating in a series system is related to the power rating while the current rating in a parallel connected system is related to the power.
(iii) There are increased losses in the line and valves in series system in comparison to parallel systems.
(iv) Insulation coordination is a problem in series system as the voltage along the line varies.
(v) The permanent fault in a line section would lead to complete shutdown in a series connected system while it would lead to only the shutdown of a converter station connected to the line section in a radial MTDC system.
(vi) The reduction in AC voltages and commutation failures in an inverter can lead to overloading of converters as current is transferred from other terminals in a parallel system.
(vii) The control and protection philosophy in a series MTDC system is a natural extension of that in a two terminals system. However, extension to parallel systems is not straight forward. It requires more communication system and also there are problems associated from commutation failures with parallel systems.
(viii) The series connection is appropriate for taps of rating less than 20% of the major inverter terminal, while parallel connection is more versatile and is expected to be used in AC systems.
Feeders
Distribution system is a part of power system, which is between distribution substations the consumer. According to design considerations, the primary distribution system is classified into three types i.e.., radial, loop and network systems.
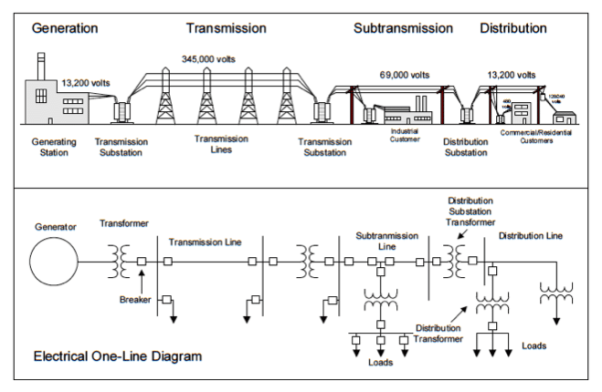
DESIGN CONSIDERATIONS OF RADIAL TYPE DISTRIBUTION FEEDER
Most distribution systems are designed as radial distribution systems as shown in figure. The radial system is characterized by having only on path between each customer and a substation. The electrical power flows exclusively away the substation and out to the customer along a single path, which, if interrupted results in complete loss of power to the customer. Radial design by far, is the most widely used form of distribution design, accounting for over ninety- nine percent of all distribution in India. Its predominance is due to two overwhelming advantages: its lower cost than the other two alternative and simple in planning, design and operation.
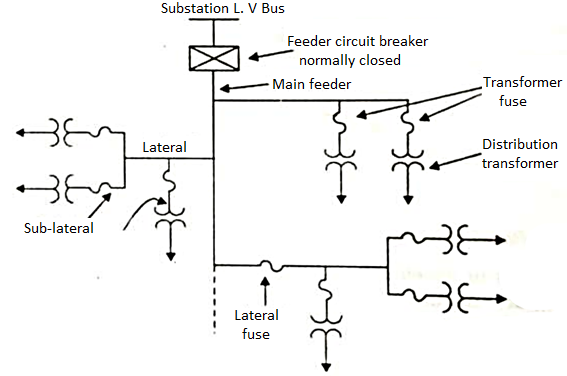
Each radial feeder serves a definite service area. Most radial feeder systems are built as networks, but operated radially by opening switches at certain points throughout the physical network (shown in fig), so that the resulting configuration is electrically radial. The planner determines the layout of the network and the size of each feeder segment in that network and decides where the open points should be for proper operation as a set of radial feeders.
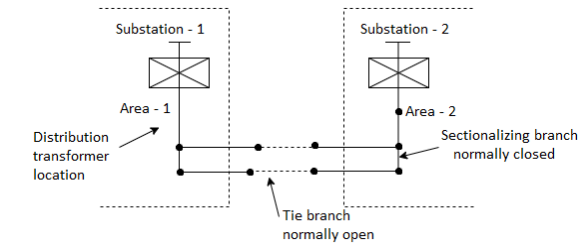
The other type of radial primary feeder with express feeder and back feed is as shown in figure below. The section of the feeder between the substation LV bus and the load center of the service area is called express feeder. From which no sub feeders or laterals will be allowed to be tapped off. The portion from load center towards the substation is called back feed portion. However ,a sub feeder is allowed to provide a back-feed towards the substation from the load center.
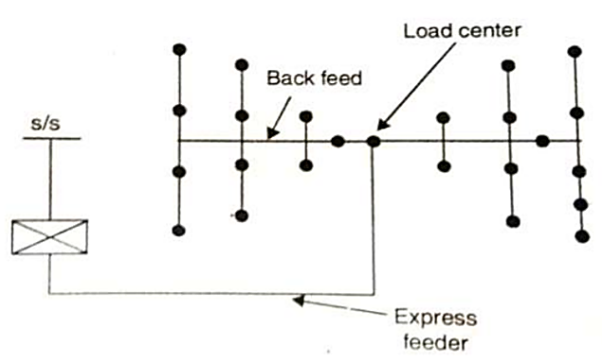
Most of the utilities in developed countries are using single and two-phase laterals to deliver power over short distances by tapping off only one or two phases of the primary feeder minimizing the amount of wire that need be strong for the short segment required to get the power in general vicinity of few customers. Each service transformer in these systems feed power into a small radial system around it. Regardless of whether it uses single-phase laterals or not, the advantages of the radial system, in addition to its lower cost, are the simplicity of analysis and predictability for performance.
Because there is only one path between each customer and the substation, the direction of power flow is absolutely certain. Equally important is that the load on any branch of the system can be determined in the most straightforward manner by simply adding up all the customer loads “downstream” from that piece of equipment. Before the advent of economical and widely available computer analysis, this was only an over helming advantage. Simple, straight forward,” back of the envelope” design procedures can be applied to the distribution system with confidence that the resulting system would work well. The simplicity of analysis and confidence that operating behavior is strictly predictable are major advantages.
Because load and power flow direction are easy to establish, voltage profiles can be determined with accuracy without resorting to exotic calculation methods; equipment capacity requirements can be ascertained at exactly fault levels, can be predicted with a reasonable degree of accuracy; and protective devices, breaker-relays and fuses can be coordinated in an absolutely assured manner, without resorting to network methods of analysis. The major drawback of radial feeder is that it is less reliable than loop or network system because there is only one path between the substation and the customer.
Thus, if any branch along this path fails a loss of power delivery results. Generally, when such failure occurs, a repair crew is dispatched to re-switch temporarily the radial pattern network, transferring the interrupted customers onto another feeder, until the damaged branch can be repaired. This minimizes the period of outages, but an outage still occurred because of the failure.
DESIGN CONSIDERATIONS OF LOOP TYPE DISTRIBUTION FEEDER
An alternative to purely radial feeder design is a loop system as shown in figure below consisting of a distribution design with two paths between the power sources (substations, service transformers) and every customer. Equipment is sized and each loop is designed so that service can be maintained regardless of where an open point might be on the loop. Because of this requirement, whether operated radially (with one open point in each loop) or with closed loops, the basic equipment capacity requirements of the loop feeder design do not change.
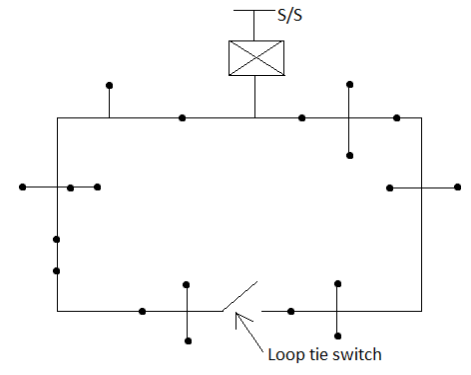
In terms of complexity, a loop type distribution system is only slightly more complicated than a radial system, power usually flows out from both sides toward the middle. Voltage drop, sizing and protection engineering are slightly more complicated than radial systems. But if designed thus and if the protection (relay-breakers and sectionalizes) is also built to proper design standards the loop system is more reliable than radial systems. Service will not be interrupted to the majority of customers whenever a segment is out of service, because there is no “downstream” portion of any loop. The major disadvantage of loop systems is capacity and cost. A loop must be able to meet all power and voltage drop requirements when fed from only one end, not both. It needs extra capacity on each end and the conductor must be large enough to handle the power and voltage drop of the entire feeder, if fed from either end. This makes the loop system inherently more reliable than a radial system, but the larger conductor and extra capacity increase cost.
DESIGN CONSIDERATIONS OF NETWORK TYPE DISTRIBUTION FEEDER
Distribution network is the most complicated; the most reliable and even in very rare cases also it is the most economical method of distributing electric power. A network involves multiple paths between all points in the network as a shown in figure below. Power flow between any two points is usually split among several paths and if a failure occurs it instantly and automatically re-routes itself. Rarely in a distribution network primary voltage-level network design is involved, in which all or most of the switches between feeders are closed so that the feeder system is connected between substations. This is seldom done because it proves very expensive and often will not work well. Instead, a “distribution network” almost always involves “interlaced” radial feeders and a network secondary system grid of electricity strong conductor connecting all the customers together at utilization voltage. Most distribution networks are underground simply because they are employed only in high density areas, where overhead space is not available.
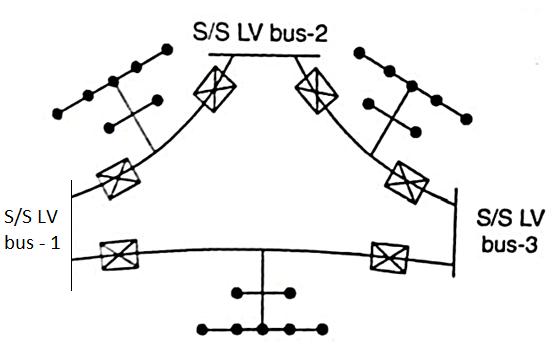
The reliability and the quality of service of the network type distribution arrangement are much higher than the radial and loop arrangements. However, it is more difficult to design and operate than the radial or loop type systems.
A distributor is a conductor from which tappings are taken for supply to the consumers. The current through a distributor is not constant because tappings are taken at various places along its length. While designing a distributor, voltage drop along its length is the main consideration since the statutory limit of voltage variations is ± 6% of rated value at the consumers’ terminals.
Key takeaways
Feeder | Distributor |
In the substance (s), the voltage magnitude is reduced to a utilization level and then the eclectic power is a distribution to the various area through the conductors which is known as feeders. | The distributor is a conductor which distributes the electrical power in particular area. |
A feeder has no tapping on it. | It has a number of tapping on me through which the electrical power is actually reached to consumer premises. |
Current through it always remains same. | The current flowing through it does not remain same. |
While designing a feeder the main consideration is its current carrying capacity. | While designing a distributor the main consideration is a voltage drop and the variation should be within + 6% of rated voltage. |
SR. No. | Equipment | Location | Function |
1 | Bus bars | Supported on post insulator or strain insulator | To receive power from the incoming circuit and to deliver power to the outgoing circuit |
2 | Surge arresters | Connected between phase conductors and ground | To discharge overvoltage surges to earth. |
3 | Isolators | Located on each side of C.T. | To provide isolation from Life parts for maintenance |
4 | Earthing switch | Mounted on the frame of the isolators | For safety purpose, it discharges the voltage on the circuit to earth |
5 | Circuit Breaker | At every switching point. At both ends of protection zones. | Switching off the part during the abnormal condition and reconnect or normal condition. |
6 | C.T. | Protective or measuring C.T. Location decided by protective zone measurement requirement | Step down current measurement protection and control. |
7 | P.T. | Located on the feeder side of C.B. | Step down voltage is for measurement, protection, and control.
|
8 | Power T/F | At generating station, the receiving station. | To set up voltage for the transmission line, to step down for feeder line. |
9 | Shunt reactors | Transmission line | During a low load, period to control voltage of long extra High voltage lines. Also, to compensate shunt capacitance of transmission line during low load period. |
10 | Shunt capacitors | Located at receiving stations and distribution substations. | To improve P.F. By compensating reactive power point. |
11 | Series capacitors | Bank located at sending and/or receiving and offline | To improve power transferability in EV long line. |
12 | Carrier equipment | Mounted above CVT or a separate structure | Use for protection and communication signals. |
13 | Switchgear | Located inside switchgear building | To provide AC power to the auxiliary station lighting system. |
14 | Station earthing system | Connected to equipment earthing and neutral point earthing | To provide low resistance earthing for discharge current from search arresters earthing switches. |
15 | Insulators | On cross across | To support conductors. |
References:
1. N.N.Rao Electromagnetic Engg. V Edition, Prentice Hall. 2005
2. Fawwaz T. Ulaby Applied Electromagnetics, Prentice Hall. 1999
3. Krauss Electromagnetic Engg. IV Edition, Tata Mc Graw Hill. 2003
4. Shevgaonkar Electromagnetic Waves, Tata Mc Graw Hill 2002
5. Matthew, N. O. Sadiku Elements of Electromagnetics, Oxford University publication, 6th edition, 2014.