Unit - 5
Three phase Induction Motor Design: Part2
The stator/rotor slot combinations and armature winding configurations with different pole pairs for DC-biased VRMs is thoroughly investigated. Firstly, the relationships among stator/rotor slots and armature winding pole pairs are based on the working principles. Then, several feasible stator/rotor slot combinations are obtained, and the electromagnetic performances are compared by the finite element analysis (FEA).
The results show that the 8-rotor-slot, 5 armature pole pair machine exhibits highest torque in rated load, but with highest torque density, the 10-rotor-slot, 4-armature pole pair machine shows highest torque in over load condition, and the 12/11 and 12/13 machine presents the minimum pulsation torque
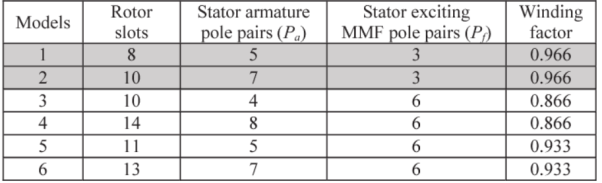
Slot/Pole Combinations and Armature Winding Factors of 12-Stator-Slot Machine
Calculations for main dimensions and stator design parameters:
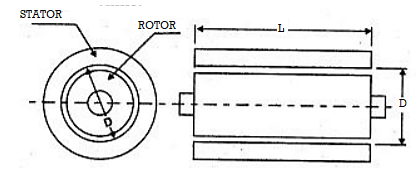
Figure 1. Main dimensions of D and L
The armature diameter D (or stator bore) and stator core length L are known as main dimensions. The quantity D 2 L is in m3 called as volume of the machine.
The size or volume of the active parts of machine is given by D 2 L which depends on two factors
- Output co-efficient Co
- Speed
Thus, obtain smallest dimensions of machine, the output co-efficient Co must be selected as high as possible. The separation of D and L from product D2L depends on ratio L/ where
D/p.
In induction motors most of the operating characteristics are decided by L/ ratio of motor. The ratio of core length to pole pitch L/
for various design features are listed in table.
Table L/ values:
Design Feature | Ratio L/ ![]() |
(1) Minimum cost | 1.5 to 2 |
(2) Good Power Factor | 1 to 1.25 |
(3) Good Efficiency | 1.5 |
(4) Good Overall design | 1 |
Generally, L/ lies between 0.6 to 2. It is shown that for best power factor the pole pitch
is given the equation

The diameter of the stator bore therefore the diameter of rotor is limited by peripheral speed. Standard constructions are employed for peripheral speeds upto 60 m/s. For normal design, the diameter should be chosen that the peripheral speed does not exceed about 30m/sec.
Stator Design:
- Number of turns in main winding:
Stator induced voltage E = 4.44 fTm Kwm.
Where Tm = number of turns in the running winding
Kwm = winding factor for the running winding
Number of turns in the running winding
= flux per pole
flux density x slots per pole x Wb
The number of turns per series pole for the main(running) winding= Tmp.
- Running winding conductors current carried by each running winding conductor
I rated = hp x 0.746 / V η cos Ø
Efficiency and power factor for single phase motors.
Area of running winding conductor Am = Imδ;whereδ is the current density in Amps/mm2
Conductor size for the running winding can be calculated as follows-
Therefore, area of running winding conductor = IRatedδ
Diameter size of running winding conductor d=
- Starting winding
The stating winding is designed for maximum torque per ampere of starting current. To calculate starting torque and current, the rotor resistance is increased by 17.5% to consider the skin effect. Therefore, total resistance in terms of main winding Rm=rsm+1.175 r’rm at 20 .
Total impedance at 20 Zm=
Auxiliary winding reactance Xla=Xlm/((Is/Ism)^2- 1)
Resistance of auxiliary winding Ra=(Rm+Zm(Is/Ism))/((Is/Ism)^2-1)
Auxiliary winding conductor section aa= TaLmta / rsa
Diameter of bare conductor =
In capacitor start method reactance of the capacitor
Xc=Xla+((Ra*Rm)/(Zm+Xlm))
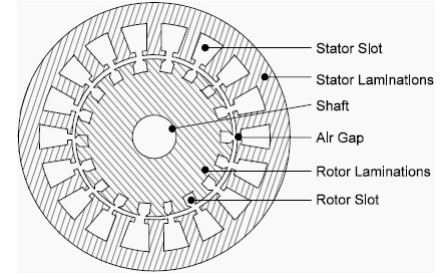
Figure 2. Stator and Rotor slot
- The induction motor has stator and rotor as its major parts. The stator is stationary part while the rotor is a rotating part. The stator of the motor consists of overlapping coils that are placed 120-degree electrically apart.
- When the stator is connected to three phase supply sources, the rotational magnetic field of constant magnitude and varying direction is produced. The flux produced in stator travels through the air gap and gets linked to rotor conductors.
- The rotor winding is short-circuited through end rings or connected to the external resistance. The linking flux of stator induces EMF in rotor circuit, and current starts to flow in the short-circuited rotor winding because of induced EMF in rotor. Due to interaction of rotor current and main flux, torque is produced, and motor starts rotating.
- In the stator the electric energy gets converted to magnetic energy. The magnetic energy travels through the air gap of the motor. The air gap increases the reluctance of the magnetic circuit.
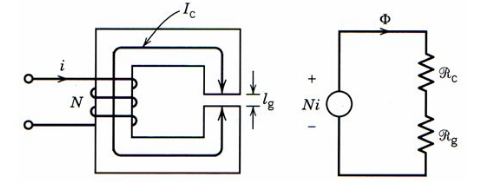
Figure 3. Equivalent Electrical circuit of the magnetic Circuit
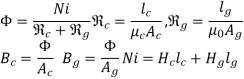
Where,
Rc and Rg is the reluctance of the magnetic and the sir gap circuit.
The air gap plays a significant role in the performance of the motor.
The length of the air-gap affects the following performance parameters of the induction motor.
Magnetizing current
Power factor
Overload Capacity
Cooling
Noise
Magnetizing Current and Power Factor:
The magnetizing current of the motor depends on specific magnetic loading and the length air gap of the machine. A large air-gap length leads to higher magnetizing current and poor power factor.
A large air-gap length increases the reluctance of the path of the magnetic flux. The reluctance of the magnetic circuit is like the resistance of the electric circuit. The reluctanceof a magnetic circuit is;
R=MMF/Φ -----(1)
Also,
R= L/μA -------(2)
MMF=RΦ
Φ =MMF/R
Φ =MMF*(μA /L) -----(3)
Where,
R = Reluctance of the magnetic circuit
MMF =Magnetomotive force(MMF=NI)
L =length of the air gap
Φ =Flux in the air gap
μ = Permeability of the magnetic material
The MMF required for producing and sending the flux through the air gap depends on the flux density and the air-gap length.
If air gap length increases, the reluctance of the magnetic circuit increases. This increase in reluctance will demand more magneto-motive force in order to produce the required flux in the motor.
In order to meet the additional requirement of MMF, the stator magnetizing current is increased. The power factor of the motor gets worsened with an increase in magnetizing stator current. The phasor diagram of the motor having small and large air gap length is shown below. The relationship between the air gap length and the power factor can be understood with the help of phasor diagram.
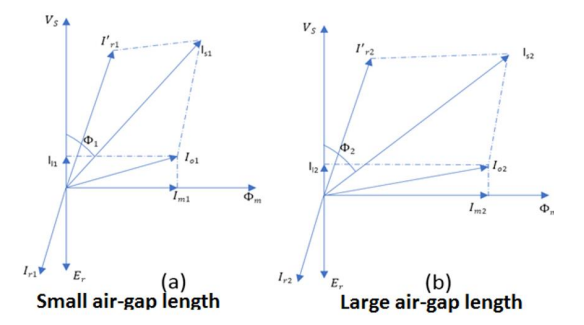
Figure 4. Small and Large air-gap length
The air gap length in fig (b) is more than the air gap in fig (a). The angle between the applied voltage and stator current is more in fig(b) than in fig(a).With increase in air gap length, more magnetizing current is required to produce the rated flux in the magnetic core, and the phase angle between the applied voltage and the magnetizing current increases. As a result, power factor becomes low.
Overload Capacity:
The leakage flux is reduced for an increase in air gap length. The flux produced in the stator winding gets almost completely coupled with the rotor winding if air gap length is more. Hence, overload capacity of large air gap length motor is more than overload capacity of the motor that has a small air-gap length. With an increase in the air-gap length, the leakage reactance decreases and overload capacity increases.
Cooling:
With an increase in air gap length, the stator and rotor are separated by large distance, hence cooling is better. The copper loss (I^2*R Loss) takes place in the stator and rotor winding and iron loss takes place in the core. The heat gets transferred easily if the motor has large air- gap. The insulation of the winding can be used of H class or F class with temperature rise limit to B class if the air gap length is more.
Noise:
The leakage flux gets reduced if air- gap length is more. Less noise is generated in motor with more air- gap length.
Tooth pulsation loss:
The tooth pulsation loss reduces in a large air- gap length motor because of small difference in the reactance of air gap.
The optimum air gap of an induction motor can be expressed by the following empirical formula.
lg = 0.2 + 2√ LD mm
Where,
Lg = Air gap Length (mm)
L = Stator core Length(Meter)
D = Internal diameter of the Stator core(Meter)
Example:
If the stator core length and diameter is 0.18 meter and 0.34 meter respectively, the air gap length between the stator and the rotor
Lg = 0.2 + 2√ LD mm
= 0.2 +2√0.18*0.34
= 0.2 +0.494
= 0.694 mm
Proper numbers of rotor slots must be selected in regard to number of stator slots otherwise undesirable effects will be found while the starting of the motor. Cogging and Crawling are the two phenomena observed due to wrong combination of number of rotor and stator slots. Also, the induction motor may develop unpredictable hooks and cusps in torque- speed characteristics or the motor may run with lot of noise.
Crawling: The rotating magnetic field produced in the air gap is non-sinusoidal and contains odd harmonics of 3rd, 5th and 7th order. The third harmonic flux will produce three times the magnetic poles compared tothe fundamental. Similarly, the 5th and 7thharmonicsproduces the poles five and seven times the fundamental respectively.
The presence of harmonics in the flux wave affects the torque speed characteristics. The Figure below shows the effect of 7th harmonics on the torque speed characteristics of three phase induction motor. The motor with presence of 7th harmonics tends to tend to run the motor at one seventh of its normal speed. The 7th harmonics will produce a dip in torque speed characteristics at one seventh of its normal speed as shown in torque speed characteristics.
Cogging: In some cases where the number of rotor slots are not proper in relation to number of stators slots the machine refuses to run and remains stationary. Under such conditions there will be a locking tendency between the rotor and stator. Such a phenomenon is called cogging.
In order to avoid such bad effects, proper number of rotor slots must be selected in relation to number of stator slots.
Also, the rotor slots will be skewed by one slot pitch to minimize the tendency of cogging, torque defects like synchronous hooks and cusps and noisy operation while running.
Effect of skewing slightly increases the rotor resistance and increases the starting torque. However, this increases the leakage reactance thereby reduces the starting current and power factor.
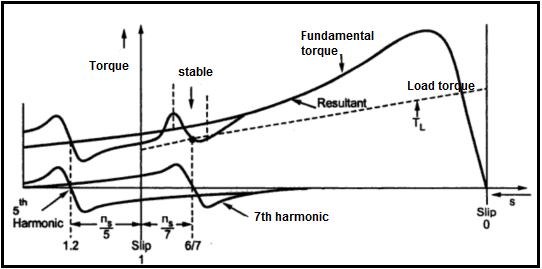
Figure 5. Torque – Speed characteristics
Selection of number of rotor slots: The number of rotor slots may be selected using the following guidelines.
(i) To avoid cogging and crawling: (a)Ss ≠ Sr (b) Ss - Sr ≠ ±3P
(ii) To avoid synchronous hooks and cusps in torque speed characteristics ≠ ±P, ±2P, ±5P.
(iii) To noisy operation Ss - Sr ≠ ±1, ±2, (±P ±1), (±P ±2)
Generally semi-closed slots or closed slots have an exceedingly small or narrow opening used for the rotor slots. In fully closed slots the rotor bars are forced to fit into the slots from the sides of the rotor.
The rotors with closed slots give better performance to the motor in the following way.
(i) As the rotor is closed the rotor surface is smooth at air gap and hence motor draws lower magnetizing current.
(ii) Reduces noise because air- gap characteristics are better
(iii) Increases leakage reactance and reduces starting current.
(iv) Over- load capacity is reduced.
From the above we see that semi-closed slots are more suitable and employed in rotors.
All the rotor bars are short circuited by connecting them to the end rings at both the end rings. The rotating magnetic field produced induces emf in the rotor bars which is sinusoidal over one pole pitch. As the rotor is short -circuited body, there is current flow because of this induced emf. The distribution of current and end rings is shown in Figure. Referring to figure considering the bars under one pole pitch, half of the number of bars and the end ring carry the current in one direction and the other half in the opposite direction. Thus, maximum end ring current may be taken as the sum of the average current in half of the number of bars under one pole.
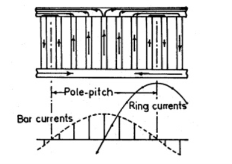
Figure 6. Currents in cage rotor bars and end rings
Maximum end ring current Ie(max) = ½ ( Number rotor bars / pole) Ib(av)
= ½ x Sr/P x Ib/1.11
Hence rms value of Ie = 1/2√2 x Sr/P x Ib/1.11
= 1/π x Sr/P x Ib/1.11
These are the types of induction motors where in rotor also carries distributed star connected 3 phase winding. At one end of the rotor there are three slip rings mounted on the shaft. Three ends of the winding are connected to the slip rings. External resistances can be connected to these slip rings at starting, which will be inserted in series with the windings which will help in increasing the torque at starting. Such type of induction motors are employed where high starting torque is required.
Number of rotor slots:
The number of rotor slots should never be equal to number of stator slots. Generally for wound rotor motors a suitable value is assumed for number of rotor slots per pole per phase, and then total numbers of rotor slots are calculated. So selected number of slots should be such that tooth width must satisfy the flux density limitation. Semi closed slots are used for rotor slots.
Number of rotor Turns:
Number of rotor turns are decided based on the safety consideration of the personal working with the induction motors. The voltage between the slip rings on open circuit must be limited to safety values. In general, the voltage between the slip rings for low and medium voltage machines must be limited to 400 volts. For motors with higher voltage ratings and large size motors this voltage must be limited to 1000 volts. Based on the assumed voltage between the slip rings comparing the induced voltage ratio in stator and rotor the number of turns on rotor winding can be calculated.
Voltage ratio Er/Es = (Kws x Tr) /((Kws x Ts)
Hence rotor turns per phase Tr = (Er/Es) (Kws/ Kwr) Ts
Er= open circuit rotor voltage/phase
Es = stator voltage /phase
Kws = winding factor for stator
Kwr = winding factor for rotor
Ts = number of stator turns/phase
The Area of rotor conductor can be calculated based on the assumed value for the current density in rotor conductor and calculated rotor current. Current density rotor conductor can be assumed between 4 to 6 Amp/mm2
Ar = Ir / δr mm2
If Ar< 5mm2 use circular conductor, else rectangular conductor, for rectangular conductor width to thickness ratio = 2.5 to 4. Then the standard conductor size can be selected like that of stator conductor.
Number of rotor Turns:
The Number of rotor turns are decided based on the safety consideration of the personal working with the induction motors. The volatge between the slip rings on open circuit must be limited to safety values.
Generally, the voltage between the slip rings for low and medium voltage machines must be limited to 400 volts. For motors with higher voltage ratings and large size motors this voltage is limited to 1000 volts.
Depending on the assumed voltage between the slip rings and comparing the induced voltage ratio in stator and rotor the number of turns on rotor winding can be calculated as
Voltage ratio Er/ Es = (Kwr x Tr) / (Kws x Ts )
Hence rotor turns per phase Tr = (Er/Es) (Kws/Kwr) Ts
Er = open circuit rotor voltage/phase
Es = stator voltage /phase
Kws = winding factor for stator
Kwr = winding factor for rotor
Ts = Number of stator turns/phase
Rotor ampere turn = 0.85 x stator ampere turn
IrTr = 0.85 x Is Ts
Rotor current Ir = 0.85 Is Ts / Tr.
References:
- K.L. Narang, A Text Book of Electrical Engineering Drawings, Reprint Edition: 1993 / 94 – Satya Prakashan, New Delhi.
- A Shanmugasundaram, G. Gangadharan, R. Palani, - Electrical Machine Design Data Book, 3rd Edition, 3rd Reprint 1988 - Wiely Eastern Ltd., - New Delhi
- Vishnu Murti, “Computer Aided Design for Electrical Machines”, B.S. Publications.
- Bharat Heavy Electricals Limited, Transformers - TMH.