UNIT 6
Satellite Communication Link Design
There are four factors related to satellite system design:
- Weight of the satellite
- Choice of frequency band
- Atmospheric propagation effects
- Multiple access technique
The major frequency bands are 6/4 GHz , 14/11 GHz and 30/20 GHz (Uplink/Downlink)
At geostationary orbit there is already satellites using both 6/4 and 14/11 GHz every 2 0 (minimum space to avoid interference from uplink earth stations)
Low earth orbit (LEO) and medium earth orbit satellite systems are closer and produces stronger signals but earth terminals need omni directional antennas.
The design of any satellite communication is based on:
- Meeting of minimum C/N ratio for a specific percentage of time.
- Carrying the maximum revenue earning traffic at minimum cost.
The calculation of power received by an earth station from a satellite is fundamental to the understanding of satellite communication.
Consider a transmitting force in free space radiating a total power Pt watts uniformly in all directions.
Such source is called isotropic.
At a distance R meters from isotropic source flux density crossing the surface
F = Pt/4π R2 (W/m 2) ----------------------(1)
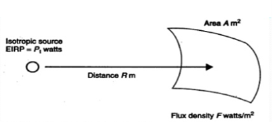
Flux density produced by an isotropic source
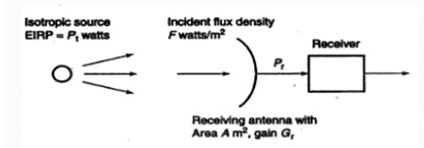
Power received by an ideal antenna with area Am2 . Incident flux density is
F = Pt/4π R2 Wim2. Received power Pt=FxA = Pt A / 4π R2 .
For a transmitter with output Pt watts driving a lossless antenna with gain Gt flux density at distance R meters is
F = PtGt/4π R2 (W/m 2)
The product PtGt is called the effective isotropic radiated power or EIRP. It describes the combination of transmitting power and antenna gain in terms of an equivalent isotropic source with power PtGt watts.
If we have an ideal receiving antenna with an aperture of A m2 we would collect Power Pr watts given by
Pr = F x A watts.
A practical antenna with physical aperture area A m2 will not deliver power as given in the above equation.
Some of the energy incident on aperture is reflected away from the antenna some is absorbed by lossy components . The effective aperture Ae is
Ae = A A A
- A = aperture efficiency of the antenna.
For Horn Antennas
- A = 90%
Thus the power received by real antenna with effective area Aem2 is
Pr = Pt Gt Ae / 4 π R 2 (watts) ------------------------(A)
A fundamental relation in antenna theory gain and area of an antenna which are related by
G = 4 π Ae / 2 ------------------------------------------(B)
Substituting B in A we get
Pr = [Pt Gt Gr / (4 π R / ) 2 ] watts
This expression is known as link equation and essential in calculation of power received in any radio link.
The term (4 π R / ) 2 is known as path loss Lp.
Collecting various factors Power received is given by
Power Received = (EIRP * Receiving antenna gain / path loss) watts
In decibel we have
Pr = EIRP + Gr – Lp--------------------------(C)
Where EIRP = 10 log (Pt Gt) dBW
Gr = 10 log 10 (4πAe/ 2) db
Lp = 10 log 10 (4 π R / ) 2 db
Equation C represents an idealized case in which there are no additional losses in the link.
In practice we need to take into account of a more complex situation in which we have losses in the atmosphere due to attenuation by oxygen, water vapour, and rain , losses in the antenna at each end of the link.
So equation C can be written as
Pr = ERIP + Gr – Lp – La – Lta –Lra dBW
Where
La = attenuation in atmosphere
Lta = losses associated with transmitting antenna
Lra = losses associated with receiving antenna
The received power Pr is commonly referred to as carrier power C
This is because most satellite links use either frequency modulation for analog transmission or phase modulation for digital systems.
In both of the modulation schemes the amplitude of the carrier is not changed when data are modulated onto the carrier so carrier power C is always equal to the received power Pr.
Noise temperature provides a way of determining how much thermal noise is generated by active and passive devices in the receiving system.
At microwave frequencies, a blak body with physical temperature , Tp degrees Kelvin generate electrical noise over a wide bandwidth.
The noise power is given by
Pn = k Tn B
Where
k = Boltzmann’s constant = 1.38 x 10 -23 J/K = -228.6 dBW/K/Hz
Tn = Noise temperature of source in K
B = noise bandwidth in which noise power is measured in Hz.
System noise temperature Ts is the noise temperature of noise source at the input of noiseless receiver which gives same noise power as the original receiver measured at the output of receiver.
Calculation
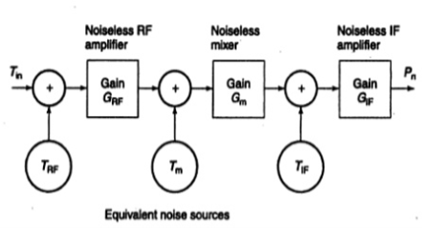
The noisy devices in the receiver are replaced by equivalent noiseless blocks with the same gain and noise generators at the input to each block such that the block produces same noise at its output as the device it replaces.
The total noise power at the output of the IF amplifier of the receiver is given by
Pn = GIF kTIF Bn + GIF Gm kTmBn + GIF Gm GRF kBn (TRF + Tin)
This equation can be written as
Pn = GIF Gm GRF[ (kTIFBn)/(GRFGm) + (kTmBn)/ GRF + (TRF + Tin)]
= GIF Gm GRF k Bn [TRF + Tin + Tm / GRF + TIF / (GRFGm)]
The single source of noise shown in above figure with noise temperature Ts generates the same noise power Pn at its output
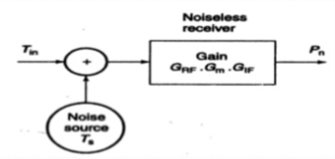
Pn = GIF Gm GRF kTs Bn
KTsBn = kBn[(Tin + TRF + Tm / GIF + TIF/ Gm GRF)]
So the system noise temperature is
Ts = [ Tin + TRF + Tm / GRF + TIF / (GmGRF)]
Noise Figure
It is used to specify the noise generated within the device
The operational noise figure is
NF = (S/N)in / (S/N) out
Noise Temperature
It is useful in satellite communication systems . It is best to convert noise figure to noise temperature.
T = To (NF – 1)
Where
NF is linear ratio
To is the reference temperature.
G/T Ratio for earth stations:
The link equation can be rewritten in terms of (C/N) at the earth stations
C/N = [ Pt Gt Gt / kTsBn] [/4π R] 2 = [ PtGt/kBn][
/4πR] 2 [Gt/Ts]
The design of any satellite communication is based on two objectives :
- Meeting a minimum C/N ratio for a specified percentage of time
- Carrying the maximum revenue earning traffic at minimum cost.
Any satellite link can be designed with very large antennas to achieve high C/N ratios under all conditions but cost is high.
The art of good system design is to reach the best compromise of system parameters that meets the specification at the lower cost.
The ground station or earth station is the terrestrial base of the system.
An earth station consists of five major subsystems:
- The antenna subsystem
- The receive subsystem
- The transmit subsystem
- The ground control equipment (GCE) subsystem
- Power subsystem
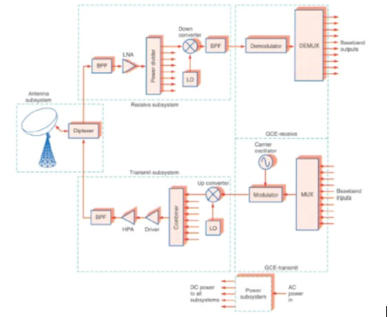
- The antenna subsystem
Antennas are present in both satellite and earth station. Satellite antennas perform two types of functions. Those are receiving of signals, which are coming from earth station and transmitting signals to one or more earth stations based on the requirement.
In other words, the satellite antennas receive uplink signals and transmit downlink signals.We know that the length of satellite antennas is inversely proportional to the operating frequency. The operating frequency has to be increased in order to reduce the length of satellite antennas. Therefore, satellite antennas operate in the order of GHz frequencies.
- Receiver subsystem
During reception, the earth station antenna receives downlink signal. This is a low-level modulated RF signal. In general, the received signal will be having less signal strength. So, in order to amplify this signal, Low Noise Amplifier (LNA) is used. Due to this, there is an improvement in Signal to Noise Ratio (SNR) value.
RF signal can be down converted to the Intermediate Frequency (IF) value, which is either 70 or 140 MHz. Because, it is easy to demodulate at these intermediate frequencies.
The function of the decoder is just opposite to that of encoder. So, the decoder produces an error free binary information by removing error correction bits and correcting the bit positions if any.
This binary information is given to base band equipment for further processing and then delivers to terrestrial network.
- Transmit Subsystem
- The binary (digital) information enters at base band equipment of earth station from terrestrial network. Encoder includes error correction bits in order to minimize the bit error rate.
- In satellite communication, the Intermediate Frequency (IF) can be chosen as 70 MHz by using a transponder having bandwidth of 36 MHz. Similarly, the IF can also be chosen as 140 MHz by using a transponder having bandwidth of either 54 MHz or 72 MHz.
- Up converter performs the frequency conversion of modulated signal to higher frequency. This signal will be amplified by using High power amplifier. The earth station antenna transmits this signal.
- GCE subsystem
The GCE in an earth station interfaces the baseband signals to transmit and receive subsystems. The receive GCE performs demodulation and de-multiplexing. The transmit GCE performs modulation and multiplexing.
- Power Subsystem
The basic dc voltage from the solar panels is conditioned in various ways.
For example it is typically passed through voltage regulator circuits before being used to power individual electronic circuits.
- The Uplink design is easier than the downlink since an accurately specified carrier power must be presented at the satellite transponder and it is often feasible to use much higher transmitters at earth stations that can be used on a satellite.
- The cost of the transmitter tends to be high with the cost of the receiving equipment in satellite communication system.
- Earth station transmitter power is set by the power level required at the input to the transponder.
- Analysis of uplink requires calculation of the power level at the input to the transponder so that the uplink C/N ratio is found.
- The link equation is used to make this calculation.
- Let (C/N ) up be the specified C/N ratio in the transponder measured in an noise bandwidth Bn Hz.
The noise power referred to the transponder input is N1p W where
Nip = k + Tip + Bs dBW
Where Tip is the system noise temperature of the transponder in dBK and Bs is in units of dBHz.
The power received at the input to the transponder Prsp where
Prsp = Pt + Gt + Gt – Lp – Lsp dBW
Where PtGt is the uplink earth station EIRP in dBW , Gt is the satellite gain in dB in the direction of uplink earth station and Lp is the path loss in dB. The factor Lsp dB accounts for all uplink losses other than the path loss .
The value (C/N) sp at the LNA input of the satellite receiver is given by
C/N = 10 log 10 [PT/(kTsBn)] = Prsp –Nsp dB
The earth station transmitter output power Pt is calculated using C/N and noise power Nsp is calculated . The received power at transponder input is given by
Prsp = N + C/N dBW
At frequencies above 10GHz propagating disturbances in the form of fading in rain causes the received power level at the satellite to fall.
This lowers the upper (C/N) ratio in the transponder which lowers the overall (C/N)o ratio in the earth station receiver.
When more than one C/N ratio is present in the link we can add the individual C/N ratios reciprocally to obtain the overall C/N ratio denoted as (C/N) o
The overall (C/N)o ratio is
(C/N) o = 1/ [1/(C/N)1 + 1/(C/N)2 + ------------]
This sometimes is referred as reciprocal C/N formula.
The C/N values must be linear ratios not decibel values.
(C/N) o = C / (N1 + N2 +………………)
In dB units
(C/N) o = C dBW -10 log 10 (N1 +N2 + ………………………..) dB
C/N ratio at the receiver always yields (C/N) o the combination of transponder and earth station C/N ratios.
Design Procedure:
- Determine the frequency band in which the system must operate. Comparative designs may be required to make the selection
- Determine the communication parameters of the satellite. Estimate any values that are not known.
- Determine the parameters of transmitting and receiving earth stations.
- Start at the transmitting earth station. Establish an uplink budget and transponder noise power to find (C/N) up in the transponder.
- Find the output of the transponder based on transponder gain or output backoff.
- Establish a downlink power and noise budget for the receiving earth station. Calculate (C/N) dn and (C/N) o for a station at the edge of the coverage zone.
- Calculate S/N or BER in baseband channel. Find the link margin.
- Evaluate the results and compare with the specific requirements. Change the parameters of the system as required to obtain acceptable (C/N)o or S/N or BER values.
- Determine the propagation conditions under which the link must operate. Calculate the outage times for the uplinks and downlinks.
- Redesign the system by changing some parameters if the link margins are inadequate. Check all the parameters so that the design can be implemented within the expected budget.
References:
- Optical Fiber Communications: Principles and Practice John M Senior
- Optical Fiber Communications by Gerd Keiser