Module 2
Aromatic systems & their molecular structure
The name ‘aromatic’ was originated from the characteristic odor or ‘aroma’ of benzene-like compounds, chemists now have a completely different method of deciding whether a compound is aromatic or not. Based on the analysis of a number of compounds with unusual resonance stabilization energies, the following characteristics have been accepted as criteria for aromaticity.
1. The molecule must be cyclic, planar with uninterrupted cloud of π electrons above and below the plane of the ring.
2. It should have 4n+2 π electrons.
Here every atom in the ring must have a p orbital and the delocalization should result in an uninterrupted cyclic cloud of π electrons above and below the plain of the ring. The German Chemist Erich Hückel was the first one to recognize that an aromatic compound must have an odd number of pairs of electrons, which can mathematically be written as 4n+2 (n = 0,1,2,3 etc). Molecules which obey these rules are aromatic and those which follow these rules partially fall in the category of anti-aromatic and non aromatic compounds.
The three general requirements for a compound to be aromatic are:
- The compound must be cyclic
- Each element within the ring must have a p-orbital that is perpendicular to the ring, hence the molecule is planar.
- The compound must follow Hückel's Rule (the ring has to contain 4n+2 p-orbital electrons).
Among the many distinctive features of benzene, its aromaticity is the major contributor to why it is so unreactive. This section will try to clarify the theory of aromaticity and why aromaticity gives unique qualities that make these conjugated alkenes inert to compounds such as Br2 and even hydrochloric acid. It will also go into detail about the unusually large resonance energy due to the six conjugated carbons of benzene.
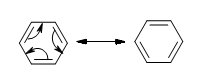
The delocalization of the p-orbital carbons on the sp2 hybridized carbons is what gives the aromatic qualities of benzene.
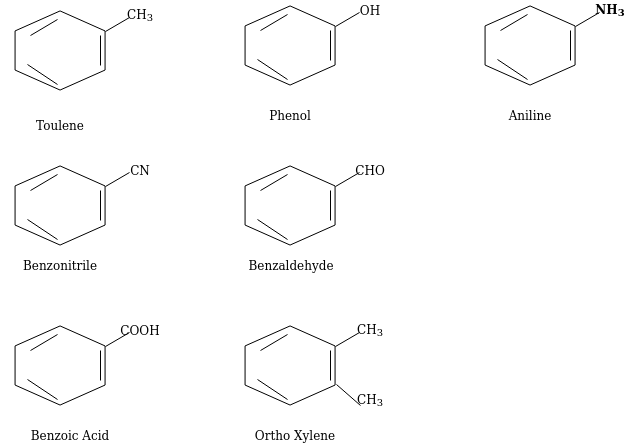
The most common aromatic compounds are derivatives of benzene (an aromatic hydrocarbon common in petroleum and its distillatres), the word aromatic occasionally refers informally to benzene derivatives, and so it was first defined. Nevertheless, many non-benzene aromatic compounds exist. In living organisms, for example, the most common aromatic rings are the double-ringed bases in RNA and DNA. An aromatic functional group or other substituent is called an aryl group.
Huckel’s Rule is used in order to estimate the aromatic qualities of any planar ring-shaped molecule in the field of organic chemistry. The supporting quantum mechanics required for the formulation of this rule was solved first by the German physical chemist and physicist Erich Armand Arthur Joseph Huckel in the year 1931.
Huckel’s rule states that only planar, fully conjugated monocyclic polyenes having 4n + 2 π electrons, where n is an integer, that is, n = 0, 1, 2, 3, 4, etc., should possess aromatic stability. An aromatic compound must be planar and contain a cyclic cloud of π electrons below and above the plane of the molecule. It contains SP2 hybridized carbon atoms and must obey the Huckel rule.
According to this rule, the ring system must have (4n+2) π electrons, where n is any whole number (0, 1, 2, 3, etc). On this basis the ring systems which have 2(n=0), 6(n=1), 10(n=2), 14(n=3) etc pi electrons are aromatic. Typical examples of aromatic compounds are benzene, naphthalene, and anthracene.
Huckel 4n + 2 Pi Electron Rule:
A ring-shaped cyclic molecule is said to follow the Huckel rule when the total number of pi electrons belonging to the molecule can be equated to the formula ‘4n + 2’ where n can be any integer with a positive value (including zero).
Examples of molecules following Huckel’s rule have only been established for values of ‘n’ ranging from zero to six. The total number of pi electrons in the benzene molecule depicted below can be found to be 6, obeying the 4n+2 𝛑 electron rule where n=1.
This rule is also justified with the help of the Pariser-Parr-Pople method and the linear combination of atomic orbitals (LCAO) method.
Generally, aromatic compounds are quite stable due to the resonance energy or the delocalized electron cloud. For a molecule to exhibit aromatic qualities, the following conditions must be met by it:
- There must be 4n + 2 𝛑 electrons present in a system of connected p orbitals (where the electrons are delocalized) belonging to the molecule.
- In order to meet the first condition, the molecule must have an approximately planar structure wherein the p orbitals are more or less parallel and have the ability to interact with each other.
- The molecule must have a cyclic structure and must have a ring of p orbitals which doesn’t have any sp3 hybridized atoms.
Other examples of aromatic compounds that comply with Huckel’s Rule include pyrrole, pyridine, and furan. All three of these examples have 6 pi electrons each, so the value of n for them would be one.
Structure and bonding of benzene: The molecular formula of benzene is C6H6. Benzene is a clear, colorless, highly flammable and volatile, liquid aromatic hydrocarbon with a gasoline-like odor. Benzene is found in crude oils and as a by-product of oil-refining processes. In industry benzene is used as a solvent, as a chemical intermediate, and is used in the synthesis of numerous chemicals. Exposure to this substance causes neurological symptoms and affects the bone marrow causing aplastic anemia, excessive bleeding and damage to the immune system. Benzene is a known human carcinogen and is linked to an increased risk of developing lymphatic and hematopoietic cancers, acute myelogenous leukemia, as well as chronic lymphocytic leukemia.
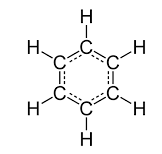
Benzene molecule is planar in shape with each C-C bond being 1.39 Å in length and each bond angle being 120°. The delocalization of the ring makes each count as one and a half bonds between the carbons which makes sense because experimentally we find that the actual bond length is somewhere in between a single and double bond. Finally, there are a total of six p-orbital electrons that form the stabilizing electron clouds above and below the aromatic ring. A molecular orbital description of benzene provides a more satisfying and more general treatment of "aromaticity". We know that benzene has a planar hexagonal structure in which all the carbon atoms are sp2 hybridized, and all the carbon-carbon bonds are equal in length. As shown below, the remaining cyclic array of six p-orbitals (one on each carbon) overlap to generate six molecular orbitals, three bonding and three anti-bonding. The plus and minus signs shown in the diagram do not represent electrostatic charge, but refer to phase signs in the equations that describe these orbitals (in the diagram the phases are also color coded). When the phases correspond, the orbitals overlap to generate a common region of like phase, with those orbitals having the greatest overlap (e.g. π1) being lowest in energy. The remaining carbon valence electrons then occupy these molecular orbitals in pairs, resulting in a fully occupied (6 electrons) set of bonding molecular orbitals. It is this completely filled set of bonding orbitals, or closed shell, that gives the benzene ring its thermodynamic and chemical stability, just as a filled valence shell octet confers stability on the inert gases.
Benzene, C6H6, is a planar molecule containing a ring of six carbon atoms, each with a hydrogen atom attached.
Benzene is hexagonal which is formed by the complete presence of six carbon atom. All of the carbon-carbon bonds have exactly the same lengths - somewhere between single and double bonds.
There are delocalized electrons above and below the plane of the ring.
The presence of the delocalized electrons makes benzene particularly stable.
Benzene resists addition reactions because those reactions would involve breaking the delocalization and losing that stability.
Benzene is represented by this symbol, where the circle represents the delocalized electrons, and each corner of the hexagon has a carbon atom with a hydrogen attached.
Bonding in Benzene:
Building the orbital model Benzene is built from hydrogen atoms (1s1) and carbon atoms (1s22s22px12py1). Each carbon atom has to join to three other atoms (one hydrogen and two carbons) and doesn't have enough unpaired electrons to form the required number of bonds, so it needs to promote one of the 2s2 pair into the empty 2pz orbital.

There is only a small energy gap between the 2s and 2p orbital, and an electron is promoted from the 2s to the empty 2p to give 4 unpaired electrons. The extra energy released when these electrons are used for bonding more than compensates for the initial input. The carbon atom is now said to be in an excited state.
Hybridization in Benzene:
Because each carbon is only joining to three other atoms, when the carbon atoms hybridise their outer orbitals before forming bonds, they only need to hybridise three of the orbitals rather than all four. They use the 2s electron and two of the 2p electrons, but leave the other 2p electron unchanged. The new orbitals formed are called sp2 hybrids, because they are made by an s orbital and two p orbitals reorganising themselves. The three sp2 hybrid orbitals arrange themselves as far apart as possible - which is at 120° to each other in a plane. The remaining p orbital is at right angles to them. This is all exactly the same as happens in ethene. The difference in benzene is that each carbon atom is joined to two other similar carbon atoms instead of just one. Each carbon atom uses the sp2 hybrids to form sigma bonds with two other carbons and one hydrogen atom. The p electron on each carbon atom is overlapping with those on both sides of it. This extensive sideways overlap produces a system of pi bonds which are spread out over the whole carbon ring. Because the electrons are no longer held between just two carbon atoms, but are spread over the whole ring, the electrons are said to be delocalized. The six delocalized electrons go into three molecular orbitals - two in each. In common with the great majority of descriptions of the bonding in benzene, we are only going to show one of these delocalized molecular orbitals for simplicity.
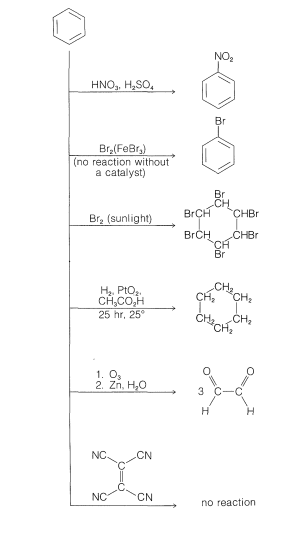
Structure and bonding of pyrrole:
Microwave spectroscopic measurements show that pyrrole is a planar molecule which is symmetrical about the y-axis, i.e. it has C2v symmetry. Recent determinations of the bond lengths and angles for pyrrole support the earlier values reported by Bak, although Cumper's values for the bond lengths of the C2—C3 and C3—C4 bonds warrant closer inspection.

Pyrrole can be considered to be a resonance hybrid of canonical structures (Ala)-(Alf). Unlike benzene, where two equivalent Kekule structures can be drawn, only one classical structure (Ala), which makes a major contribution to the resonance hybrid, can be written for pyrrole. Although the zwitterionic structures (Alc)-(Alf) make smaller contributions to the resonance hybrid, it is evident that the electron density at the a- and ß-carbon atoms should be significantly greater than predicted from the classical structure. The N—C2, N—C5, and C3—C4 bonds should be shorter, and the C2—C3 and C4~C5 bonds longer than normal single and double bonds. The lone pair on nitrogen is in the p orbital so it is involved in the 6 pi-electron aromatic system. Hence pyrrole is not very nucleophilic and is only weakly basic at nitrogen. Looking at the HOMO of pyrrole the lobes are much bigger at the 2- and 5- positions, this indicates that the reactions are most likely to take place at these positions.
Attack at C2 is also most favoured if you consider resonance forms as there are three resonance forms of the cation available, whereas there are only two resonance forms following attack at C3 so attack is less favoured at C3.