Module 05
Polymers
5.1 Introduction: Definition- Polymer:
Polymer chemists study large, complex molecules that are built up from many smaller units. They study about the smaller building blocks combine, and create useful materials with specific characteristics by manipulating the molecular structure of the monomers/polymers used, the composition of the monomer/polymer combinations, and applying chemical and processing techniques that can to a large extent, affect the properties of the final product. Polymer chemists are unique within the chemistry community because their understanding of the relationship between structure and property spans from the molecular scale to the macroscopic scale.
It is a long molecule formed by joining together of thousands of small molecular units by chemical bonds. Polymers are giant molecules also called macromolecules that are essential to our existence. They are important chemicals in our bodies in plants (starch, cellulose) and in our everyday lives (fibers, plastics, elastomers). Polymers are made by transforming small molecules into molecules with very large molecular weights. Although the chemical properties of polymers are similar to those of analogous small molecules, their physical properties are quite different. Every polymer has its own characteristics, but most polymers have the following general properties:
Polymers can be very resistant to chemicals.
Polymers can be both thermal and electrical insulators.
Generally, polymers are light in weight with varying degrees of strength.
Polymers can be processed in various ways to produce thin fibers or intricate parts.
Polymer is a molecule formed by joining of thousands of smaller molecular units together by chemical bonds. A chemical process that leads to the formation of polymer is known as polymerization. Degree of polymerization: The number of repeat units monomeric units available in the polymer is known as degree of polymerization.
Functionality: The number of bonding sites or reactive sites or functional groups present in the molecule. Ex: The double bond in vinyl monomers (CH2 = CHX) can be considered as a site for two free valencies. When the double bond is broken, two single bonds become available for combination.
H2C=CHX → CH2 – CHX
1. When the functionality of monomer is two bifunctional linear (or) straight chain polymer is formed. Ex: (a)vinyl monomers (b)adipic acid (c)hexamethylene diamine (d)terephthalic acid(e)ethylene glycol (f )amino acid Example for polymer: HDPE (high density polythene)
2. When the functionality of monomer is three (tri-functional), threedimensional net work polymer is formed. Ex: phenol, glycerol Examples for polymers : Urea formaldehyde, phenol formaldehyde.
Polymerization: The process in which the simpler molecules combine together to form very large molecule having high molecular weight is known as polymerization. The molecule is known as polymer. The different ways of doing polymerization are –
By opening a double bond
Ex:
NCH2 = CH2 (CH2 – CH2)n
Ethane polythene
NCH2=CH (CH2 – CH)n
Cl Cl
Vinyl chloride polyvinyl chloride
Types of polymerization:
Polymers can be synthesized by the following polymerization processes:
- Addition polymerization or chain polymerization
- Condensation or step or step growth polymerization
III. Copolymerisation
Addition polymerization:
The addition polymerization is the process in which the liniking together of monomer molecules by a chain reaction is observed. Polymer synthesized by addition polymerization has the same empirical formula as that of monomer. No molecule is evolved during polymerisation and the polymer is an exact multiple of the original monomeric molecule.
Condensation polymerization:
An intermolecular reaction involving two different bifunctional reactants with affinity for each other and taking place through repeated condensation reaction is known as condensation polymerization.
Copolymerisation:
Addition polymerisation involving a mixture of two or more suitable or compatible monomers gives a copolymer and the process is known as copolymerization. A reaction in which a mixture of two or more monomers is allowed to undergo polymerisation is known as copolymerization. The polymer is known as copolymer.
E.g.: Copolymerisation of styrene and methyl methacrylate
Properties of Polymers-Molecular weight:
Polymer properties depend of their structure and they are divided into classes according to their physical basis. Many physical and chemical properties describe how a polymer behaves as a continuous macroscopic material.
Mechanical properties
The bulk properties of a polymer are those most often of end-use interest. These are the properties that dictate how the polymer actually behaves on a macroscopic scale. Polymers are usually described as viscoelastic materials, which emphasizes their intermediate position between viscous liquids and elastic solids. An ideal linear elastic solid obeys Hooke’s law, i.e. stress is proportional to strain. An ideal viscous liquid obeys Newton’s law, i.e. stress is proportional to the rate of change of strain.
Chemical properties
The attractive forces between polymer chains play a large part in determining the polymer’s properties. Because polymer chains are so long, they have many such interchain interactions per molecule, amplifying the effect of these interactions on the polymer properties in comparison to attractions between conventional molecules. Different side groups on the polymer can lend the polymer to ionic bonding or hydrogen bonding between its own chains. These stronger forces typically result in higher tensile strength and higher crystalline melting points.
Molecular Weight:
Molecular weight is the average weight of the molecules that make up a polymer and gives an indication of the length of the polymer chains.
The polymerization process is subject to variation so there is no single chain length, there is actually a wide range of lengths, so when we discuss molecular weight, we really mean the average molecular weight of the material. This average is found by measuring samples of the material as it is produced.
Unless they have been purified, synthetic polymers have a distribution of molecular weights. Different methods of measuring the molecular weight yield different types of averages. Two of the most important are the number-average and the weight-average molecular weights. Suppose that you have a set of values {x1, x2, . . ., xn} for which you calculate an average value. If the probability of each value occurring are given by {P1, P2, . . ., Pn} then the average is given by the sum below:
∑i=0 ∞ Pixi
Numerical problems on molecular weight:
a. To Find:
(a) The number-average molecular weight
(b) The degree of polymerization for the given polypropylene material b. Given:
Molecular Weight Range | xi | wi |
8,000–16,000 | 0.05 | 0.02 |
16,000–24,000 | 0.16 | 0.10 |
24,000–32,000 | 0.24 | 0.20 |
32,000–40,000 | 0.28 | 0.30 |
40,000–48,000 | 0.20 | 0.27 |
48,000–56,000 | 0.07 | 0.11 |
c. Assumptions:
The given data is accurate; the material (polypropylene) is pure.
Solution:
Number-average molecular weight:
Molecular Weight Range | Mean Mi | xi | xiMi |
8,000–16,000 | 12,000 | 0.05 | 600 |
16,000–24,000 | 20,000 | 0.16 | 3200 |
24,000–32,000 | 28,000 | 0.24 | 6720 |
32,000–40,000 | 36,000 | 0.28 | 10,080 |
40,000–48,000 | 44,000 | 0.20 | 8800 |
48,000–56,000 | 52,000 | 0.07 | 3640 |
Mn = xiMi
= 33,040 g/mol
Degree of polymerization:
For polypropylene, the repeat unit molecular weight,
m = 3(AC ) + 6(AH )
= (3)(12.01 g/mol) + (6)(1.008 g/mol)
= 42.08 g/mol
DP = Mn / m
= 33,040 /42.08 g/mol
= 785
Effect of heat on polymers (glass transition temperature):
Glass transition temperature is described as the temperature at which 30–50 carbon chains start to move. At the glass transition temperature, the amorphous regions experience transition from rigid state to more flexible state making the temperature at the border of the solid state to rubbery state. It is also said that at this temperature the free volume (gap between the molecular chains) increases by 2.5 time. Glass transition temperature is represented by Tg and is a property of the amorphous materials or the amorphous portion of a semicrystalline materials. When the ambient temperature is below Tg, the molecular chains of amorphous materials are frozen in place and behave like solid glass. Plastic materials with flexible backbone show lower Tg, whereas plastic materials whose molecular structure is stiff, rigid, and inflexible show a higher Tg. Glass transition temperature helps determine various flexible and rigid applications for a material.
Polymers are hard and brittle like glass, due to the lack of mobility below Glassy state (Tg)
Polymers are soft and flexible like rubber due to some mobility above Glassy State (Tg)
The physical and polymer properties of the polymer also changes above the Glassy State (Tg)
Melting point also known as melt temperature is the critical temperature above which the crystalline regions in a semi crystalline plastic are able to flow. Semi crystalline polymers begin to soften above Tg, however, they do not demonstrate fluid behavior until the Tm range is achieved. In general, Tm for a semi crystalline polymer is higher than its Tg. At temperature above Tg but below Tm, there is a “rubbery region,” where the material can exhibit large elongations under relatively low load. Plastic materials are made up of uneven chain lengths and require different amount of energy to move which means that Tg or Tm for amorphous and semi crystalline polymers is not one definite temperature but a range of temperature during which all the chains start to move and experience complete flow. At temperature above melting point, semi crystalline plastics exhibit a rapid phase change from solids to viscous liquids that can be molded or given shapes. Even though, amorphous material starts transitioning into leathery region at Tg and continues to soften over a wide range of temperature.
Viscoelasticity:
Viscoelasticity is the property of materials that exhibit both viscous and elastic characteristics when undergoing deformation. Viscous materials, like water, resist shear flow and strain linearly with time when a stress is applied. Elastic materials strain when stretched and immediately return to their original state once the stress is removed.
- Viscoelasticity is the behavior of materials with both fluid and elastic properties at the same time.
- Viscoelasticity is caused by temporary connections between fiber-like particles.
- Polymers always show a visco elastic behavior because they consist of long molecules able to make temporary connections with their neighbors.
- Next to polymers examples of other materials with visco elastic properties are spaghetti, shag (tobacco) and a pile of worms moving through each other.
Types of Visco Elasticity:
(i) Linear Visco Elasticity
(ii) Non-Linear Visco Elasticity
Linear Visco Elasticity:
Visco elastic materials are those for which the relationship between stress and strain depends on time. Linear visco elastic materials are those for which there is a linear relationship between stress and strain (at any given time). Linear visco elasticity is a theory describing the behavior of such ideal materials. Strain-time curves for various constant stresses are shown in the following figure for a linear visco elastic material. At any given time, say t1 , the strain is proportional to stress, so that the strain due to 3o at the t1 is three times the strain due to o same time.
Non-Linear Visco Elasticity:
Nonlinear visco elasticity is when the function is not separable. It usually happens when the deformations are large or if the material changes its properties under deformations.
An elastic material is a special case of a visco elastic material will fully recover to its original state on the removal of load.
Effect of temperature on visco elastic behavior:
The secondary bonds of a polymer constantly break and reform due to thermal motion. Application of a stress favors some conformations over others, so the molecules of the polymer will gradually "flow" into the favored conformations over time. Because thermal motion is one factor contributing to the deformation of polymers, visco elastic properties change with increasing or decreasing temperature. In most cases, the creep modulus, defined as the ratio of applied stress to the time-dependent strain, decreases with increasing temperature. Generally speaking, an increase in temperature correlates to a logarithmic decrease in the time required to impart equal strain under a constant stress. In other words, it takes less work to stretch a visco elastic material an equal distance at a higher temperature than it does at a lower temperature.
Conducting Polymers:
Conducting polymers have been widely utilized in biomedical applications due to their conductivity, compatibility and low-cost process ability. In addition, compared with traditional metal or semiconductor materials, conducting polymers are more biocompatible. These advantages make conducting polymers more attractive to bioengineering researchers. The mechanism of electrical conductivity of conducting polymers is based on the transmission of polarons and bipolarons. The synthesis of conducting polymers can be accomplished by electrochemical polymerization, which is more straightforward than traditional chemical synthesis. In this chapter, several applications of conducting polymers in biomedical engineering are illustrated with examples. The applications include the use of conducting polymers in biosensors, neural electrodes, drug delivery and bioactuators. The chemical origins of such a remarkable difference in the material properties between various types of polymers can be readily -bonds and hence a charge oncerationalized. Traditional polymers, such as polyethylene or polypropylene, are made up of essentially created on any given atom on the polymer chain is not mobile. The -conjugation in polymers, however, confers the required mobility to charges that are created on the polymerpresence of an extended backbone (by the process of doping) and make them electrically conducting. One problem is that, due to the presence of this extended conjugation along the polymer backbone, the chains are rigid and possess strong inter chain interactions resulting in insoluble and infusible materials. These conjugated polymers, hence, lacked one of the most important and useful properties of polymers, namely their ease of process ability. More recently, however, it was demonstrated that when lateral substituent’s were introduced, even conjugated polymers can be made soluble (hence, process able) without significant loss in their conductivity. One other problem that plagued this field from its inception, is the inherent instability of these polymers (especially, in the doped form) to ambient conditions. Today, conducting polymers that are stable even in the doped form have been prepared. We shall highlight some specific examples of such systems and discuss some of their potential applications.
Classification-Thermoplastic and Thermosetting polymers:
Thermoplastic polymer: A thermoplastic is a resin that is solid at room temperature but becomes plastic and soft upon heating, flowing due to crystal melting or by virtue of crossing the glass transition temperature (Tg). Upon processing, usually via injection-molding or blow-molding-like processes, thermoplastics take the shape of the mould within which they are poured as melt, and cool to solidify into the desired shape. The significant aspect of thermoplastics is their reversibility, the ability to undergo reheating, melt again, and change shape. This allows for additional processing of the same material, even after being prepared as a solid. Processes such as extrusion, thermoforming, and injection molding rely on such resin behavior. Some common thermoplastic materials include polyethylene (PE), polycarbonate (PC), and polyvinyl chloride (PVC).
Thermosetting Polymer: A thermosetting resin, or thermosetting polymer, is generally a liquid material at room temperature which hardens irreversibly upon heating or chemical addition. When it is placed in a mould and heated, the most solidifies into the specified shape, but this solidification process includes the formation of certain bonds, called cross links, that hold the molecules in place and change the basic nature of the material, preventing it from melting. As a result, a there most, as opposed to a thermoplastic, cannot return to its initial phase, rendering the process irreversible. There most, upon heating, become set, fixed in a specific form. During overheating, there most tend to degrade without entering a fluid phase. Processes such as compression molding, resin transfer molding, pultrusion, hand lay-up, and filament winding depend on thermosetting polymer behavior. Some common there most include epoxy, polyimide, and phenolic, many of which are significant in composites.
Properties:
Property | Thermoplastics Polymer | Thermosetting Polymer |
Molecular Structure | Linear polymer: weak molecular bonds in a straight-chain formation | Network polymers: high level of crosslinking with strong chemical molecular bonds |
Melting point | Melting point lower than the degradation temperature | Melting point higher than the degradation temperature |
Mechanical | Flexible and elastic. High resistance to impact (10x more than thermosets). Strength comes from crystallinity | Inelastic and brittle. Strong and rigid. Strength comes from crosslinking. |
Polymerization | Addition polymerisation: repolymerised during manufacture (before processing) | Polycondensation polymerisation: polymerised during processing |
Microstructure | Comprised of hard crystalline and elastic amorphous regions in its solid state | Comprised of thermosetting resin and reinforcing fibre in its solid state |
Size | Size is expressed by molecular weight | Size is expressed by crosslink density |
Solubility | Can dissolve in organic solvents | Do not dissolve in organic solvents |
Service temperature | Lower continuous use temperature (CUT) than thermosets | Higher CUT than thermoplastics |
Compounding of plastic:
Compounding is the best method for changing the characteristic of engineered thermoplastics. The final compound product is a blend of plastics and additives. Plastic compounding companies NY can help you create beautiful and strong products. With the help of melt blending plastics, it is possible to change characteristics, such as:
- Physical
- Electrical
- Thermal
- Aesthetic
All compounding begins with polymers or base resins. Different resin systems exist, and so you must choose the proper one with correct characteristics for your final product. Once a base resin is picked, it is time to determine the specific additives, reinforce, or fillers that will be incorporated into the compounded plastic. This process allows the final product to be pre colored or flame retardant. It can also make plastic more or less conductive, while also strengthening final parts. There are several steps taken when creating specialty compounding. In the end, pellets are sent to customers for sheet extrusion or injection molding.
Compounding consists of preparing plastic formulations by mixing and/or blending polymers and additives in a molten state to achieve the desired characteristics. These blends are automatically dosed with fixed set points usually through feeders/hoppers. It is mostly a blend of copolymers such as ABS, SAN, SMA etc. with additives such as anti-oxidants, UV-stabilizers and other value adding agents and sometimes a strengthening component is added such as glass fiber.
Fabrication of plastic by Compression:
The quantum of solid waste is ever increasing due to increase in population, developmental activities, changes in life style, and socio-economic conditions, Plastics waste is a significant portion of the total municipal solid waste (MSW). It is estimated that approximately 10 thousand tons per day (TPD) of plastics waste is generated i.e. 9% of 1.20 lakh TPD of MSW in the country. Plastics are made from limited resources such as petroleum, and huge advances are being made in the development of technologies to recycle plastic waste among other resources. Plastics are non biodegradable, synthetic polymers derived primarily from petro-fossil feedstock and made-up of long chain hydrocarbons with additives and can be molded into finished products. These polymers are broken in presence of suitable catalyst, into monomers such as ethylene, propylene, vinyl, styrene and benzene. These monomers are then chemically polymerized into different categories of plastics. Plastics are generally categorized as Thermoplastics and There most Plastics. Thermoplastics can be heated up to form products and then if these end products are re-heated, the plastic will soften and melt again. These include PET, HDPE, LDPE, PP, PVC, PS etc. There most plastics can be melted and formed, but once they take shape after they have solidified, they stay solid and, unlike thermoplastics cannot be re-melted. These include Multilayer and Laminated Plastics, Bakelite, Polycarbonate, Melamine, Nylon etc. Mechanical recycling methods to make plastic products and feedstock recycling methods that use plastic as a raw material in the chemical industry have been widely adopted, and awareness has also grown recently of the importance of Thermal recycling as a means of using plastics as an energy source to conserve petroleum resources. The world's annual consumption of plastic materials has increased from around 5 million tons in the 1950s to nearly 100 million tons today. Plastics constitute approximately 3-7% of municipal waste. Presently, municipal garbage disposal departments burry the plastics along with other materials in landfill without even recognizing its ill effects. Municipal solid waste in India contains 1-4% by weight of plastic waste. Looking at the volume of plastic waste generated in India, there is urgent need for proper plastic waste management. This work is centre on plastic waste management via compression molding. Compression molding is a well known technique to develop variety of products. It is a closed molding process with high pressure application Solid plastic waste can be recycled by the process of compression molding and this will not only reduce environmental pollution as a result of plastic waste but it will also lead to production of useful plastic materials for both home and industrial use.
Injection: Transfer and Extrusion molding:
Steps involved in the Injection Molding:
The various stages of the injection molding process are carefully considered when analyzing part design, tool creation and efficient production of molded plastic products. There are a lot of factors and configurations which we won’t touch on here but the basic process is the same. Let’s start with the basics.
STEP 1: THE MOLD CLOSES
STEP 2: INJECTION
(The heated plastic is injected into the mold. As the melt enters the mold, the displaced air escapes through vents in the injection pins and along the parting line. Runner, gate and vent design are important to insure the mold is properly filled.)
STEP 3: COOLING
(Once the mold is filled the part is allowed to cool for the exact amount of time needed to harden the material. Cooling time is dependent on the type of resin used and the thickness of the part. Each mold is designed with internal cooling or heating lines where water is cycled through the mold to maintain a constant temperature)
STEP 4: PLASTICIZING THE RESIN
(While the part cools, the barrel screw retracts and draws new plastic resin into the barrel from the material hopper. The heater bands maintain the needed barrel temperature for the type of resin being used.)
STEP 5: EJECTION
(The mold opens and the ejector rod moves the ejector pins forward.
The part falls and is captured in a bin located below the mold.)
STEP 6: REMOVING THE RUNNER AND PACKAGING
Transfer Molding: Transfer molding is similar to compression molding; however, the material is first placed in a transfer chamber prior to entering the mold. As in compression molding, thermo sets that are cross-linked with heat are used for transfer-molding applications. Multiple cavities can be used within transfer molding since the material is entering the mold after the mold is closed. Since runners and sprues are present, shear is created. This facilitates heat needed for cross linking and flow to the cavities. Transfer-molding machines are also generally positioned with the molds opening vertically.
Since the mold is closed and clamped prior to the material entering the mold, there is no presence of flash with transfer molded parts. The dimensions of the final parts are very accurate due to the flow of the polymer being gated. Another advantage is that the cure time is faster since there is the presence of shear flow, which creates heat. Inserts can also be used to create more complex parts than can be created by compression molding.
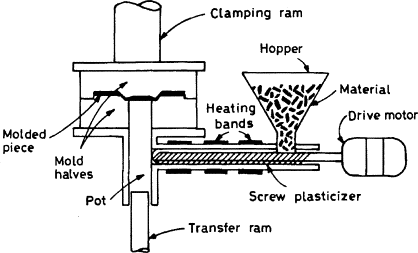
Advantages:
Shorter production cycles for higher weight parts
Transfer molding offers shorter production cycle times than traditional molding techniques, such as compression molding, as the compound preparation and product finishing time is greatly reduced. This means there is less cutting and flash. Cure times are reduced since the rubber enters the mould cavity at a higher and even temperature, and can thus begin to cure more quickly.
Tighter dimensional tolerance
The overall process also allows for much tighter tolerances, leading to more complex parts, which is difficult to achieve with compression molding. Given that the mould is not held open by surplus material spilling out of the cavity parting line, any excess holds the plunger open from the pot, therefore not affecting the actual part being produced.
Provides better uniformity
The pot and plunger design allows for more standardization and lower costs of tooling, and the process is more consistent than Compression molding, with less variables. The fact that the mould is closed before accepting the shot means that the parts are more dimensionally consistent across a production run.
Reduced tooling lead times compared to a full Injection tool
In relation to Injection Molding, Transfer Molding tooling offers much shorter lead-times with less ancillary features required. This means that the production run is ready faster with far less tooling costs.
More accurate and consistent than compression molding
Transfer molding allows for much sharper edges, since rubber enters the cavity at near the curing temperature. This vastly reduces any tendency for “backrinding”, which can be a risk with Compression molding. This occurs when rubber cures unevenly in a mould, and the action of expansion, cure and shrink tears off pieces of rubber around the split line of a mould. This is countered by increasing the width of the split line in a Compression tool, but greatly increases the overall deflashing necessary as a consequence. Transfer Molding generally eliminates this issue, and thus allows for sharper split lines.
Disadvantages:
More expensive tooling than a Compression mould
Due to the more complex nature of the design of the moulds, tooling investment can be somewhat higher.
Slower production cycle than an injection tool
The Transfer molding process is usually slower than an injection tool, sometimes limiting the overall production rate, as changeover times can be somewhat extended.
Manual handling of the piston can be a problem
The skill level is often proportionately higher and for larger parts or tools, manual handling can become somewhat of an issue.
Extrusion molding: Extrusion molding (also known as plastification extruding) is a process that the stack of powders or the green body in die is pushed out to assume another form of green body or other final product under pressure. The cold extruding process is applied to mixtures of metal powders and organic binders, and extruding is performed at low temperatures (40–200 °C) to form the green body. The processes include material preparation, preprocessing, extruding, cutting, and reforming. The porous products can be obtained after drying, pre-sintering, and sintering of the extruded green body. It is an effective way to produce a long porous tube with a small diameter. The pretreatment of a mixture under pressure involves making full contact between the plasticizer and particle surfaces, to remove the gas inclusion and finally to ensure uniform density. Plasticizers have a large effect on a material’s properties. Therefore, certain requirements are needed for them, including that there should not be any reaction with porous materials during sintering, and that they should be removable, sticky, and have great pore-forming ability. The plasticizers in common use are olefin, amylum, and polythene alcohol. Powders will be subjected to pressure from the side wall, friction from either the powder and the wall or the extrusion shaft and the wall, in addition to the normal compression from the extrusion shaft. The key factors affecting the properties of green body extrusion are the types of powders, the particle shape and size, the plasticizer type and content, the precision of the mold, the pressure from extrusion, the extrusion speed, and the preheating temperatures. The selection of the preheating temperature depends on the optimal plastic used for the plasticizer at the selected temperature. The extrusion speed can be determined experimentally, and it is closely related to the particle size, shape, extrusion ratio, fluidity, extrusion force, and plasticizer. Higher extrusion speeds may cause the green body to crack.
Preparation, Properties and uses of PMMA and Kevlar:
PMMA (Polymethyl Methacrylate):
Preparation:
This is an important thermoplastic resin. It is obtained by polymerization of methyl methacrylate which is an ester of methyl acrylic acid, CH2=C(CH3)COOH, in presence of acetyl peroxide or hydrogen peroxide. It is an acrylic polymer.
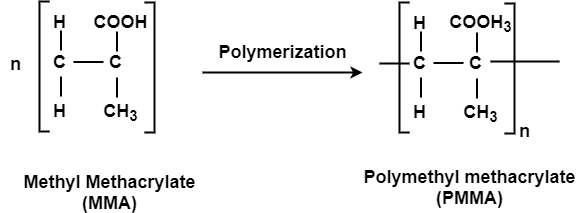
Properties:
(i) It is hard, fairly rigid material with a high softening point of about 130-14rfC.
(ii) It becomes rubber like at a temperature above 6oC.
(iii) It has outstanding shape-forming properties due to wide span of temperature from its rigid state to viscous.
(iv) It has high optical transparency.
(v) It has high resistance to sunlight and ability of transmission light accurately.
Uses:
They are used for making lenses, optical parts of instruments, air craft, light fixture, artificial eyes, wind screen, bone splints, decorative articles etc. PMMA is found in paint and used in window glasses.
Kevlar:
Preparation:
It is aromatic polyamide or poly aramide resin. It is prepared by poly condensation reaction between aromatic dichloride and aromatic diamines.
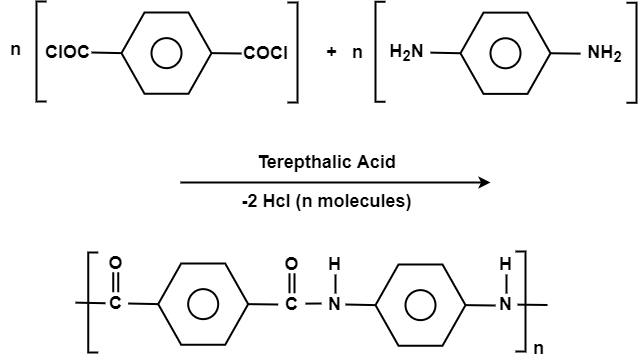
Properties:
(i) It is exceptionally strong.
(ii) It is high stability and flexibility.
(iii) Due to the delocalized bonding which causes benzene rings to be inflexible that is they posses very high rigidity.
(iv) There is high electron density in chains of Kevlar
Uses:
(i) Aerospace and Aircraft industry.
(ii) They are used in making ropes, cables, protective clothing, bullet proof vests etc.
(iii) They are useful in making motorcycle helmets.
(iv) Used in car parts. E.g. Brakes, tyres, clutch linings etc.