Unit - 4
Aromatic hydrocarbons
In organic chemistry, the aromatic qualities of any planar ring-shaped molecules are estimated by the Huckel’s Rule. The quantum mechanics was supported by the formulation of this rule which was initially solved by the German physical chemist and physicist Erich Armand Arthur Joseph Hickel in the year 1931.
The Hickel 4n + 2 Pi Electron Rule
The cyclic molecules that are ring shaped, follow Hickel rule when the total number of pi electrons belonging to the molecule can be equated to the formula ‘4n + 2’ where n is any integer with a positive value (including zero).
Examples of molecules following Hickel’s rule have only been established for values of ‘n’ ranging from zero to six. The total number of pi electrons in the benzene molecule depicted below can be found to be 6, obeying the 4n+2 𝛑 electron rule where n=1.
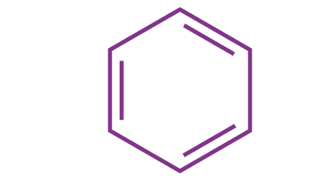
Therefore, the aromaticity of the molecule benzene is established as it obeys the Huckel rule.
This rule is also justified with the help of the Pariser-Parr-Pople method and the linear combination of atomic orbitals (LCAO) method.
The resonance energy or the localised electron cloud provide stability to the aromatic compounds. For a molecule to exhibit aromatic qualities, the following conditions must be met by it:
- There must be 4n + 2 𝛑 electrons present in a system of connected p orbitals (where the electrons are delocalized) belonging to the molecule.
- In order to meet the first condition, the molecule must have an approximately planar structure wherein the p orbitals are more or less parallel and have the ability to interact with each other.
- The molecule must have a cyclic structure and must have a ring of p orbitals which doesn’t have any sp3 hybridized atoms.
Other examples of aromatic compounds that comply with Huckel’s Rule include pyrrole, pyridine, and furan. All three of these examples have 6 pi electrons each, so the value of n for them would be one.
All arenes have general formula [CnH2n - 6y]. Where y is number of benzene rings and n is not less than 6.
Arenes are planar and cyclic. They undergo replacement rather than addition reactions.
Aromaticity or aromatic character: The characteristic behaviour of aromatic compounds is known as aromaticity. Aromaticity is because of extensive delocalisation of p-electrons in planar ring system. Huckel (1931) explained aromaticity on the basis of following rule.
For aromaticity the atom can be planar, cyclic system having delocalised (4n + 2) Π electrons where n is an integer equal to 0, 1, 2, 3,------.
Thus, the aromatic molecules have delocalised electron cloud of 2,6,10 or 14 p electrons.
For example: 4n +2 = 6; 4n = 4; n = 4/4 = 1
n = 1
n= 2 n = 3
Carbocations, are compounds that possess trivalent positively charged carbon when we consider a stabilised carbocation like tert-butyl carbocation, this concept becomes true, but, if the carbocation moiety is contained in a cyclic, conjugated system having 4n+2 pi electrons, the carbocation may be stable enough even to isolate as a salt.
a) A particularly effective example of aromatic carbocation is the cycloheptarienyl (tropylium) carbocation, this cation is similar to benzene and cyclopentadienyl anion, it has a six-pi benzene electron system. Again, the electron count is properly obtained by counting 2 electrons per pi bond and zero electrons for a carbocation centre (vacant 2p AO) in any canonical structure.

b) This cation is isolated as a salt with many counter anions that also includes tetrafluoroborate anion. It is stable in aqueous solution.
c) An isolable aromatic carbocation can be prepared in the highly strained cyclopropenyl system. Note that in the case of a cyclopropenyl system there is only one BMO, so that the aromatic system contains 2 electrons (4n+2, with n = 0).

d) The circle mnemonic and the resulting display of MOs for the cyclopropenyl system is illustrated below:
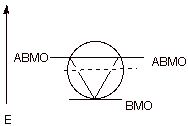
e) Stable aromatic anions and cations having 10, 14, and many higher electron count systems have also been prepared.
Carbanions and carbocations may also show aromatic stabilization. Some examples are:
Considering its ring strain the three membered ring cation has 2π-electrons and is stable, Cyclopentadiene is as acidic as ethanol, reflecting the stability of its 6 π-electron conjugate bases. Salts of cycloheptatrienyl cation (tropylium ion) are stable in water solution, again reflecting the stability of these 6 π-electron cations.
Heterocyclic compounds with suitable examples
Heterocyclic compounds such as pyridine, fyran, thiophene and pyrrole are all aromatic since each one of them is planar and has cyclic system of 6n electron which is completely delocalised over the entire range
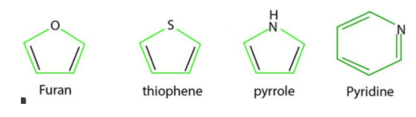
In Pyridine, each of the 5sp2 hybridised carbon atoms and the sp2 hybridised nitrogen atom has a p orbital perpendicular to the plane of the ring. Each of these atoms contributes one n electron thereby producing a single cyclic n-cloud containing 6n electrons. The lone pair of electrons on the nitrogen atom is present in a sp2 orbital which is being in the plane of the ring does not contribute towards the aromatic sextet
In Furan and Thiophene, one of the lone pairs of electrons on the sp2 hybridised heteroatom is present in a p orbital perpendicular to the plane of the ring, it contributes two n electrons while the other four p-orbitals of the two double bonds contribute one electron n each thereby the total to 6 electrons.
Pyrrole has only one lone pair which is present in a p-orbital perpendicular to the ring. Therefore , it contributes two n electrons while the fourp orbitals of the two double bond contribute one n electron thereby bringing the total to six electrons.
All the three five membered heterocycles have a single electron cloud containing 6 electrons and hence are aromatic in nature, this theory of six electrons explaining the aromatic character of cyclic compounds is called aromatic sextet theory and the six n electrons are called aromatic sextet
Annulenes: completely conjugated monocyclic polyenes containing an even number of carbon atoms are called annulenes, their general formula is (CH=CH) n where n=2,3,4…. Etc
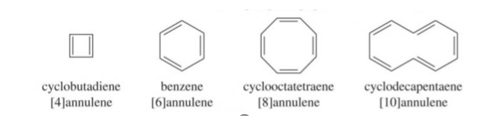
A reaction in which one or more hydrogen of the benzene ring are replaced by other monovalent atoms or group is called a substitution reaction
1. Halogenation: Benzene reacts with chlorine and bromine in the presence of Lewis acid as AlCl3, FeCl3, FeBr3 as catalyst and in the absence of light to form chlorobenzene and bromobenzene
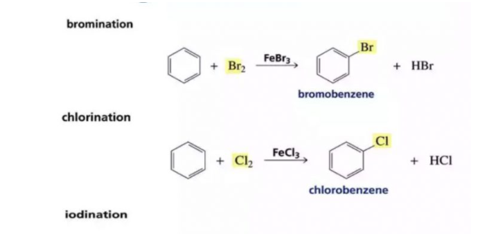
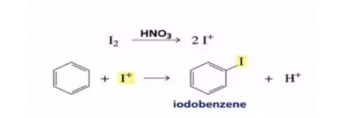
If excess of electrophilic reagent is used, two or more or even all the hydrogen atoms of benzene ring may be replaced by the electrophilic.
Benzene on treatment with excess of Cl2 in the presence of anhydrous AlCl3 in the dark yield hexachlorobenzene
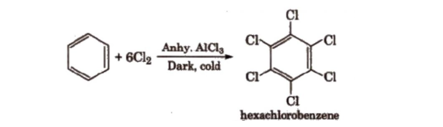
The function of lewis is to carry the halogen to the aromatic hydrcarbons, In addition to iron and aluminium halides , iodine and iron filling have been usd as halogen carrier
Chlorobenzene is formed when benzene is treated with chlorine in presence of iron, under these conditions, iron forst reacts with chlorine to form ferric chloride which then act as a catalyst. Fluorinationof arenes is too vigorous to be of any practical use.
2. Sulphonation: The process of replacement of a hydrogen atom of an arene by a sulphonic acid group (-SO3H) is called Sulphonation. It is usually carried out by treating an arene with fuming sulphuric acid or oleum or chlorosulphuric acid.
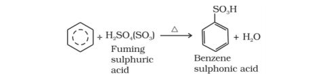
3.Nitration: The process of replacement of a hydrogen atom of an arene by the nitro(-NO2) is called Nitration, it is usually carried out by treating an arene with a mixture of conc HNO3 and conc H2SO4
4. Friedel-crafts reaction
It is of two types
a) Friedel crafts alkylation: when benzene of its homologue is treated with an alkylhalide, in the presence of anhydrous aluminium chloride as catalyst, it forms an alkylbenzene.
Friedel-Crafts Alkylation involves the replacing of aromatic proton with an alkyl group, this is achieved by the electrophilic attack on the aromatic ring with the assistance of a carbocation. It is a method of generating alkylbenzenes using alkyl halides as reactants.

The Friedel-Crafts alkylation reaction proceeds via a three-step mechanism.
Step 1
Electrophilic carbonation is formed, when an alkyl halide reacts with The Lewis acid catalyst (AlCl3).
Step 2
Cyclohexadienyl cation is formed as an intermediate, when carbocation proceeds to attack the aromatic ring. The aromaticity of the arene is temporarily lost due to the breakage of the carbon-carbon double bond.
Step 3
The deprotonation of the intermediate leads to the reformation of the carbon-carbon double bond, restoring aromaticity to the compound. This proton goes on to form hydrochloric acid, regenerating the AlCl3 catalyst.
b) Friedel Crafts acylation: On treatment with a carboxylic acid chloride or the anhydride in the presence of anhydrous aluminium chloride, benzene forms acylbenzene
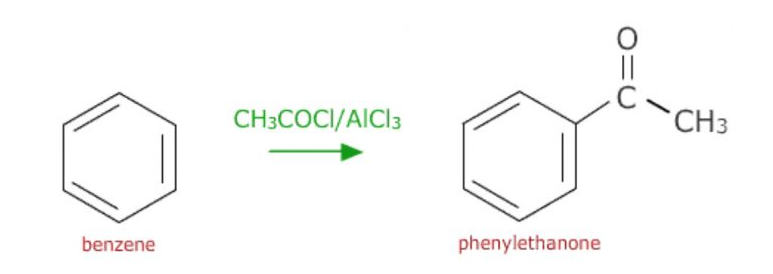
The Friedel-Crafts acylation reaction includes the adding of the acyl group to the aromatic ring. This is achieved by the involvement of an acid chloride (R-(C=O)-Cl) and also a Lewis acid chloride catalyst like AlCl3. In the acylation reaction, the aromatic ring is changed into a ketone.
Mechanism
Friedel-Crafts acylation’s proceed through a four-step mechanism.
Step 1
The reaction takes place between the acyl halide and the Lewis acid catalyst (AlCl3), resulting in the formation of a complex, and the acyl halide loses a halide ion, forming an acylium ion which is stabilized by resonance.

Step 2
The acylium ion (RCO+) performs an electrophilic attack on the aromatic ring, however the aromaticity is lost as the complex is being formed.
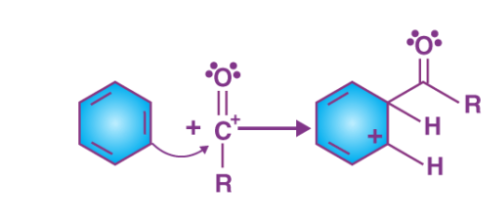
Step 3
The aromacity to the ring is restored as the intermediate complex is now deprotonated, the proton attaches itself to a chloride ion (from the complexed Lewis acid), forming HCl. The AlCl3 catalyst is now regenerated
Step 4
The catalyst that is regenerated attacks the carbonyl oxygen, hence the liberation of ketone takes place by the addition of water to the products that were formed in step3. This step can be illustrated as follows.
Thus, at the end of Friedel-Crafts acylation the require acylbenzene is formed
In an electrophilic aromatic substitution reaction, the nature of the substituent which is present on the benzene ring affects the rate and regioselectivity (relative position) of the reaction. A substituent (-X) is said to be activating if the rate of electrophilic aromatic substitution of the substituted benzene (C6H5X) is faster than benzene. The electrophilic substitution rate of reaction is deactivated by the substituent, when the rate of the reaction of the substituted benzene (C6H5X) is slower than benzene. Relative rate of nitration:
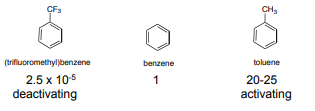
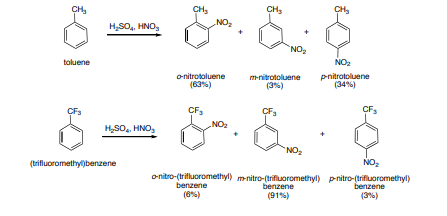
If the direction of the incoming electrophile is assisted by the substituent (-X) it is is said to be an ortho-para to itself. For example if a substituent (-X) becomes a meta director if it assists the incoming electrophile to meta position to itself
Substituents can be divided into either electron-denoting or electron -withdrawing and can alter the electron density of the aromatic ring through:
1. Inductive effects: it is the ability of a substituent to donate or withdraw electron density through σ-bonds due to electronegativity differences and bond polarities of a functional group
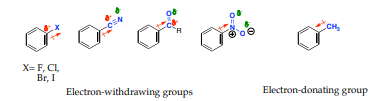
2. Resonance effects: It is the ability of a substituent to donate or withdraw electrons through non-bonding pairs of electrons or overlap π-bonds (conjugation).

Key takeaway
The aromatic hydrocarbons are “unsaturated hydrocarbons which have one or more planar six-carbon rings called benzene rings, to which hydrogen atoms are attached”. Many aromatic hydrocarbons contain a benzene ring (also referred to as an aromatic ring). The benzene ring is stabilized by resonance and the pi electrons are delocalized in the ring structure
Aromatic Hydrocarbons are circularly structured organic compounds that contain sigma bonds along with delocalized pi electrons. They are also referred to as arenes or aryl hydrocarbons.
References:
1. Graham Solomons T. W., Fryhle, Craig B., Snyder Scott A, Organic Chemistry, Wiley Student Ed, 11th Edition (2013)
2. Jonathan Clayden, Nick Greeves, Stuart Warren, Organic Chemistry, 2nd Edition, Oxford Publisher, 2014.
3. Dhawan, S.N., Pradeep’s Organic Chemistry, (Vol. I and II), Pradeep Publications