Unit - 3
Lipids
3.1.1 What are Lipids?
Lipids are hydrocarbon-containing compounds that are essential to the structure and function of living cells. Lipids include fats, oils, waxes, certain vitamins (such as A, D, E, and K), hormones, and the non-protein portion of the cell membrane.
Lipids are non-polar and so insoluble in water, but they are soluble in non-polar solvents such as chloroform.
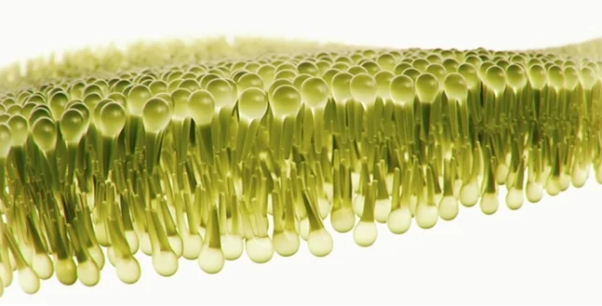
Human lipid bilayer - 3D Rendering.
3.1.2 What do Lipids Consist of?
Lipids are mostly made up of reduced hydrocarbons, which make them an efficient type of energy storage because when digested, the hydrocarbons oxidise and release enormous amounts of energy. A triglyceride, an ester made up of glycerol and three fatty acids, is the type of lipid found in fat cells for this purpose.
3.1.3 Where do Lipids Come From?
Excess carbohydrates in the diet are converted to triglycerides in the endoplasmic reticulum, which involves the creation of fatty acids from acetyl-CoA in a process known as lipogenesis. In animals and fungi, most of these functions are handled by a single multifunctional protein, whereas bacteria use numerous enzymes. Some unsaturated fatty acids, such as omega-3, cannot be produced in mammalian cells and must thus be obtained through the diet.
Acetyl-CoA also plays a role in the mevalonate pathway, which produces a variety of isoprenoids, including essential lipids like cholesterol and steroid hormones.
3.2.1 Fats and oils:
Butter, suet (beef fat), lard (pig fat), and fish oils are examples of animal fats used by humans. Olive oil, peanut (groundnut) oil, coconut oil, cottonseed oil, sunflower seed oil, soybean oil, safflower oil, rape oil, sesame (gingelly) oil, mustard oil, red palm oil, and corn oil are all important vegetable oils. Fats and oils have the highest calorie density per gramme of any diet, yet they are devoid of protein and minerals. Only butter and fish-liver oils include vitamin A or D, while red palm oil does contain carotene, which is converted to vitamin A in the body. Margarine contains vitamins A and D. Vitamin E, a fat-soluble antioxidant, is found in varying levels in all natural fats and oils.
Triglycerides, chemical molecules containing any three fatty acids coupled with a molecule of glycerol, are the most common components of fats and oils. A fatty acid is said to be saturated when there are no double bonds present, and unsaturated when there are one or more double bonds present (see the section Essential nutrients: Lipids). Butter and lard, for example, have a high percentage of saturated fatty acids and are solid at room temperature. Liquid oils, such as sunflower, safflower, and corn oils, have a high percentage of unsaturated fatty acids. The food industry uses hydrogenation to transform unsaturated oils into saturated solid fats that are more resistant to rancidity. Hydrogenation, on the other hand, results in the creation of trans-fatty acids. Saturated fatty acids appear to have some of the same negative effects on blood cholesterol as these.
In the diet, a small group of fatty acids is required. They are found in body structures, particularly inside and around cells, and cannot be generated in the body from other fats. Because it can be converted to other essential fatty acids, linoleic acid is the most significant of these fatty acids. Linoleic acid is a polyunsaturated fatty acid with two double bonds. It is an important fatty acid that also helps to decrease cholesterol levels in the blood. Many seed oils, such as corn, sunflower, cottonseed, and safflower oils, contain moderate to high amounts of linoleic acid. Some margarines (polyunsaturated margarines) are made with a combination of oils that contain a moderate amount of linoleic acid.
3.2.2 Understanding Fats and Oils
Fats and oils are organic compounds made up of the atoms carbon (C), hydrogen (H), and oxygen (O) organised into molecules, similar to carbohydrates. There are many different types of fats and oils, as well as a variety of terminology and concepts that are related with them, all of which are discussed more below.
- Triglycerides
As illustrated in Figure, triglycerides are another chemical name for the most prevalent type of fat present in the body, indicating that they are normally made up of three (tri) fatty acids and one molecule of glycerol (glycerine is another name). (The emulsifiers mono and diglycerides contain one and two fatty acids, respectively.)
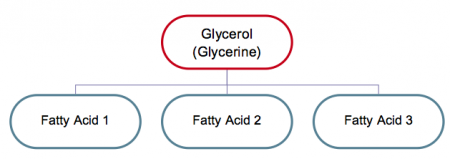
Figure. Composition of fats (triglycerides)
- Fatty Acids
The fatty acids in each type of fat or oil are distinct. The consistency of the fat or oil is determined by the type of the fatty acid. Stearic acid, for example, is the most abundant fatty acid in beef fat, while linoleic acid dominates seed oils. Depending on the number of atoms in the molecule, fatty acids are classified as short, medium, or long chain.
Because each fatty acid softens as its melting point is achieved as the temperature rises, some fat melts gradually. Fats that melt suddenly have fatty acids of the same or comparable kind with melting temperatures that are within a restricted range. Coconut fat is an example of such a fat: it is solid one second and liquid the next.
The properties of three fatty acids are shown in Table 6.
Table: Characteristics of Fatty Acids | ||
Type Of Fatty Acid | Melting Point | Physical State (at room temperature) |
Stearic | 69°C (157°F) | Solid |
Oleic | 16°C (61°F) | Liquid |
Linoleic | -12°C (9°F) | Liquid |
- Rancid
Fat that has gone rancid is referred regarded as rancid. When exposed to air and heat, the fat develops an undesirable flavour. If kept out of the refrigerator, unsalted butter, for example, will quickly get rancid, especially in warm climes.
- Oxidation/Antioxidants
Fats become rancid as a result of oxidation (exposure to air) over time. This is exacerbated by the presence of certain metals, such as copper. This is why copper pans are never used to fry doughnuts!
Some oils contain natural antioxidants, such as tocopherols (vitamin E is one kind), but these are often destroyed during the processing. As a result, synthetic antioxidants are added to prevent rancidity. Synthetic antioxidants like BHA and BHT are routinely utilised by fat makers.
- Saturated/Unsaturated
The degree to which the carbon atoms in a fatty acid molecule are linked or bonded (saturated) to hydrogen atoms is referred to as saturated or unsaturated. The number of double bonds is one method of fatty acid classification.
- 0 double bonds: saturated fatty acids. Stearic acid is a typical long-chain saturated fatty acid.
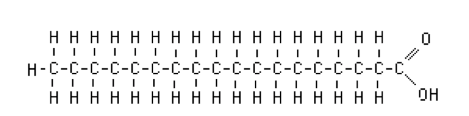
Figure. Stearic acid
- Monounsaturated fatty acids have one double bond. A typical monounsaturated fatty acid is oleic acid.
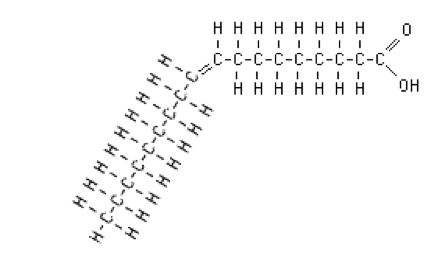
Figure. Oleic acid.
- Polyunsaturated fatty acids have two or more double bonds. Linoleic acid is a polyunsaturated fatty acid that is found in many foods.
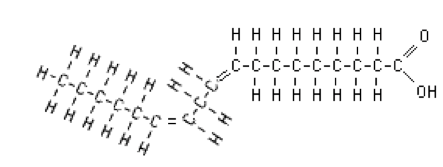
Figure. Linoleic acid.
Saturated fat is a type of dietary fat. Saturated fats have been linked to an increased risk of heart disease for many years; nevertheless, there have been studies suggesting the opposite, and the research is far from convincing. The common consensus is that the less saturated fat consumed, the better for one's health. Low saturated fat levels, on the other hand, make it difficult for fat manufacturers to create oils that can withstand the high temperatures required for deep-frying. Technology's solution has been hydrogenation. The topic of hydrogenation will be covered later in the chapter.
Saturated fat can be found in a variety of foods, including:
• Coconut, palm, and palm kernel oils
• Animal foods (such as beef, chicken, lamb, pork, and veal)
• Products derived from milk (like butter, cheese, and whole milk)
• Liquid lard
• Condensation
Unsaturated fat might also be found in your diet. Saturated and trans fats (see below) can be replaced with unsaturated fats to help lower cholesterol levels and minimise the risk of heart disease. Omega-3 and omega-6 fatty acids, which are commonly referred to as "good" fats, are also found in unsaturated fat. Using Canada's Food Guide, select foods high in unsaturated fat as part of a well-balanced diet.
Even though unsaturated fat is a “good fat,” having too much in your diet may lead to having too many calories, which can increase your risk of developing obesity, type 2 diabetes, heart disease, and certain types of cancer.
Unsaturated fats are divided into two categories:
• Monounsaturated fat, which can be found in avocados and other fruits and vegetables.
o Seeds and nuts (like cashews, pecans, almonds, and peanuts)
o Oils derived from plants (like canola, olive, peanut, safflower, sesame, and sunflower)
• Polyunsaturated fat, which can be found in the following foods:
o Fish that are fatty (like herring, mackerel, salmon, trout and smelt)
o Omega-3 fatty acids
o Seeds and nuts (like cashews, pecans, almonds and peanuts)
o Oils derived from plants (like canola, corn, flaxseed, soybean and sunflower)
- Hydrogenation
Simply described, hydrogenation is the addition of hydrogen gas to an oil or fat to change its melting point. The hydrogen injected forms a connection with the available carbon, converting liquid oil to solid fat. This is useful because it allows lipids to be used in a variety of ways. Consider the various temperature settings in a bakery where fat must be usable, as well as the various climatic circumstances encountered in bakeries.
- Trans Fat
A chemical process known as "partial hydrogenation" produces trans-fat. This is the process of turning liquid oil into solid fat. Trans fat, like saturated fat, has been shown to elevate LDL or "bad" cholesterol levels, thereby increasing your risk of heart disease. Trans fat, like saturated fat, reduces HDL or "good" cholesterol. A low level of HDL cholesterol is also linked to an increased risk of heart disease.
Until recently, the majority of trans fat in the average Canadian diet came from:
• Butter and margarine (especially hard margarines)
• Foods that have been commercially fried
• Bakery items prepared with partially hydrogenated oils and fats, such as shortening, margarine, or oils containing partially hydrogenated oils and fats (including cakes, cookies, crackers, croissants, doughnuts, fried and breaded foods, muffins, pastries, and other snack foods)
The Food and Drug Administration's (FDA) regulations specify exactly what information must be printed on a label. One element of key nutrition information that must be reported in a nutrition facts table is the amount of trans fat in a food. The open textbook Nutrition and Labelling for the Canadian Baker has more information on a nutrition facts table and labelling details.
- Emulsification (Emulsified Shortenings)
Emulsification is a method of combining ordinarily incompatible components (such as oil and water) into a stable material. Emulsifiers are chemicals that can help with this. In egg yolks, there exist natural emulsifiers such as lecithin. Emulsifiers are monoglycerides and diglycerides that have been added to a variety of hydrogenated fats to improve their capacity to:
• Create a fine, consistent framework.
• Absorb a high proportion of sugar
• Hold a high percentage of liquid in suspension
Emulsified shortenings are excellent for cakes and icings, but not for deep frying.
- Stability
The capacity of a shortening to have a longer shelf life is referred to as stability. It applies to deep-frying fats in particular, where a smoke point (see below) of 220°C to 230°C (428°F to 446°F) denotes a fat with a high level of stability.
- Smoke Point
The temperature at which fat begins to smoke is known as the smoke point. As the fat breaks down, the smoke point will decrease.
- Fat Breakdown
Hydrolysis is the chemical reaction of a material with water, and it is the scientific term for fat breakdown. Fatty acids are isolated from their glycerol molecules during this process and accumulate in the fat over time. The fat takes on a disagreeable taste when their concentration reaches a particular degree, and continuing use of the fat will result in a foul flavour. The moisture that is at the base of this issue originates from the frying of the product. This is why, between batches of frying things like doughnuts, it's a good idea to turn off the fryer or set it to "standby." Excess flour on the product or particles coming off the product are two more causes of fat breakdown.
3.3.1 Hydrogenation
Solid fats are ideal for many food applications as well as some commercial ones. The main constituents in many shortenings and margarines are hydrogenated (hardened) oils. The advent of a successful process for converting low-melting unsaturated fatty acids and glycerides to higher-melting saturated products led to the development of margarine and shortening products. The technique involves adding hydrogen to double (unsaturated) bonds in the presence of a catalyst. By adding hydrogen to oleic or linoleic acid (or their acid radicals in glycerides), which are generally liquid at room temperature, stearic acid or the acid radical can be formed.
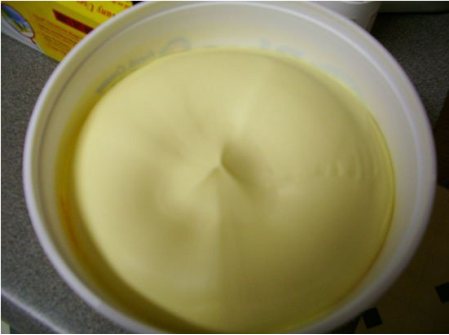
In Europe, this hydrogenation technology was only lightly used; the process's greatest potential application lay in the United States, where a massive supply of cottonseed oil, a by-product of the Southern cotton industry, awaited innovations that would allow it to be converted into a plastic fat. In the early 1900s, the hardening of cottonseed oil gave birth to the shortening industry. The use of practical hydrogenation extended to all countries where liquid oils are used to make margarines and shortenings.
3.3.2 Hydrogenation reactions
In commercial usage, hydrogenation is commonly done in a steel pressure-reaction tank with strong agitation or hydrogen dispersion with a narrow range of catalyst concentration (approximately 0.05 to 0.10 percent finely split nickel suspended on kieselguhr, or diatomaceous earth). The normal temperature and pressure ranges are 100 to 200 degrees Celsius (212 to 392 degrees Fahrenheit) and air pressure to 42 kilogrammes per square centimetre, respectively. These parameters can be tweaked to make the hydrogenation reaction more selective, allowing hydrogen to be added to the linolenic (three double bonds) and linoleic (two double bonds) acid radicals before the oleic (one double bond) acid radicals. If the conditions are right, the most unsaturated fatty acid groups are the easiest to hydrogenate and hence react first with the hydrogen. In the hydrogenation of vegetable oils, copper-containing catalysts are extremely selective. Higher temperatures and pressures are used to decrease the reaction time and use partially spent catalyst that would otherwise be wasted if very hard fats with minimal levels of unsaturation are sought and selectivity is unimportant. The hot oil is filtered after hydrogenation to remove the metallic catalyst, which can then be reused or recovered.
3.3.3 Isomerization reactions
Another process occurs during the catalytic treatment: isomerization (molecular structure rearrangement) of unsaturated fatty acid radicals to generate isooleic, isolinoleic, and related groups. These isomers contribute to the hardening effect by having higher melting points than natural acids. Natural oils have a cis unsaturation, which means hydrogen atoms are on one side of a plane that cuts through the double bond and alkyl groups are on the other. Some unsaturation is changed to the trans configuration during hydrogenation, with similar groups on opposite sides of the plane. The melting point of the trans isomers is substantially greater than that of the normal cis form. There is a migration of double bonds throughout the chain as some of the unsaturation is changed to the trans conformation. As a result, isomers of oleic acid can be made with the double bond anywhere between carbon atoms 2 and 17. The melting point of many of these isomerized acids is higher than that of natural oleic acid. Infrared analysis can be used to quantify the changes that occur during hydrogenation.
3.3.4 Hydrogenation of Unsaturated Fats and Trans Fat:
The lipid hypothesis emerged in the late 1970s, claiming that ingesting saturated fats causes higher LDL (Low Density Lipoprotein), also known as "bad cholesterol." This causes coronary heart disease, which is the constriction and hardening of arteries that leads to a heart attack. Fats were finally divided into two groups: "good fats" and "unhealthy fats." Healthy fats were thought to be unsaturated, whereas unhealthy fats were thought to be saturated.
The lipid hypothesis was determined to be invalid in a meta-analysis of 72 research including approximately 103,052 persons. “Contrary to existing guidelines, our systematic analysis showed no evidence that saturated fat raises the risk of coronary disease, or that polyunsaturated fats have a cardiac preventive effect,” the Meta-Analysis concluded. 1st Dietary fats are essential for human health. They help keep cells healthy, aid in brain growth, aid in the absorption of fat-soluble vitamins, and cushion organs from harsh assault. Saturated, unsaturated, and trans fats, to name a few, are all types of fat.
Because of their molecular form, saturated fats are solid at normal temperature. The term "saturated" refers to a sp3 carbon chain with all of its sp3 orbitals bound to hydrogen atoms. As a result, the term "saturated" was coined. Hydrogen is "saturated" in it. Saturated fats have a chain-like structure that allows them to stack exceptionally efficiently at room temperature, forming a solid. Because the sp2 carbons are trigonal planar, rather than tetrahedral (sp3 carbons) as they are in saturated fats, unsaturated fats are not linear. This results in a distinct molecular form. Fat molecules will not stack properly as a result of this structural shift, resulting in fats that are liquid at room temperature. Because butter is mainly saturated fat, it solidifies at room temperature. Olive oil is an unsaturated fat since it is liquid at room temperature. Through hydrogenation processes, an unsaturated fat can be converted to a saturated fat.
Hydrogenation Reaction
The relatively simple hydrogenation reaction can convert unsaturated fatty acids to saturated fatty acids. Remember that an alkene (unsaturated) becomes an alkane when hydrogen is added to it (saturated). The following is a simple hydrogenation reaction:

Alkene plus hydrogen yields an alkane
"Polyunsaturated" is a term used to describe vegetable oils. This simply indicates the presence of several double bonds. The hydrogenation reaction can transform liquid vegetable oils into solids. This process "hardens" margarines and shortenings, turning them solid or semi-solid.
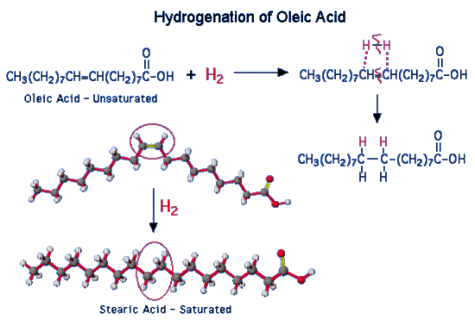
Figure: Hydrogenation of an oleic fatty acid
Partially hydrogenated vegetable oils are now partially saturated, and their melting point rises to the point where a solid is present at room temperature. The degree of hydrogenation of unsaturated oils determines the product's final consistency. What happened to the product's healthiness when it was transformed from unsaturated to saturated fats?
Trans Fat
The formation of trans fats during the hydrogenation process is a major health problem. Trans fats are formed as a result of a side reaction with the hydrogenation catalyst. This occurs when a cis isomer of an unsaturated lipid transforms to a trans isomer of the unsaturated fat. Isomers are compounds with the same chemical formula but distinct chemical bonds. A cis isomer has the hydrogens on the same side of the sp2 double bound carbons. The activation energy for converting the cis isomers of unsaturated fat to a trans isomer of unsaturated fat is obtained due to the extra energy from the hydrogenation process. One of the hydrogens is positioned on the opposite side of one of the carbons. The double bonded carbons take on a trans configuration as a result of this. Trans fats are not recognised by the human body.
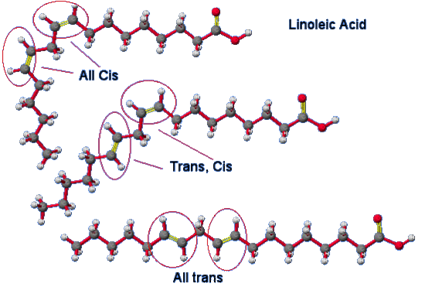
Although trans fatty acids are chemically "monounsaturated" or "polyunsaturated," they are considered so different from the cis monounsaturated or polyunsaturated fatty acids that they cannot be legally designated as unsaturated for purposes of labeling. The majority of trans fatty acids created by the partial hydrogenation process are now categorised as saturated fats, despite the fact that they are chemically still unsaturated.
The main disadvantage is that trans-fat raises "bad" LDL cholesterol while lowering "good" HDL cholesterol, though not to the same extent as saturated fat. Margarine, baked goods like doughnuts and Danish pastry, deep-fried foods like fried chicken and French-fried potatoes, snack chips, imitation cheese, and confectionary fats all include trans-fat.
3.4.1 Definition:
Soaps are an important element of keeping people's health and hygiene in check. Soaps are necessary for cleaning dirt and grease from many surfaces, including the skin. Soaps are commonly used in bathing, cleaning, and washing, as well as other home tasks.
Soap is currently a necessary everyday item that plays an important role in daily life. But, how do you make soap? Saponification is the process of creating soap. The soap-making process, also known as saponification, is explained in length and in a straightforward manner here.
The process of creating soap is known as saponification. Soaps are long-chain fatty acid potassium or sodium salts. The ester reacts with an inorganic base to produce alcohol and soap during saponification.
It happens when triglycerides are treated with potassium or sodium hydroxide (lye) to form glycerol and fatty acid salt, often known as "soap."
3.4.2 Determination of saponification value:
The saponification value is the number of mg of potassium hydroxide required to neutralise the fatty acids formed when 1 g of the substance is completely hydrolyzed.
A 50-mL burette should be used for titration in the process described, because the volume of hydrochloric acid (0.5 mol/l) VS used in the blank titration is exactly 35.5 mL when the concentration of ethanolic potassium hydroxide is exactly 40 g/l.
Recommended procedure
In a flask with a capacity of about 200 mL, place about 2 g of the test substance, accurately weighed, or the quantity stated in the monograph, and add 25 mL of potassium hydroxide/ethanol, TS1. Add 1 mL of phenolphthalein/ethanol TS and titrate the excess alkali with hydrochloric acid (0.5 mol/l) VS. Heat in a boiling water bath for 30 minutes, or the time specified in the monograph, frequently rotating the contents of the flask; immediately add 1 mL of phenolphthalein/ethanol TS and titrate the excess alkali with hydrochloric acid (0.5 mol/l) VS. To titrate the sample, record the number of mL of hydrochloric acid (0.5 mol/l) VS needed (a). Without the material to be tested, repeat the procedure and record the number of mL of hydrochloric acid (0.5 mol/l) VS necessary for neutralisation (b). Use the following formula to calculate the saponification value:
Saponification value
3.4.3 Determination of Saponification Value of Fats and Oils
Principle
When fat is hydrolyzed with an alkali, fatty acid salts (commonly known as soap) and glycerol are formed. Saponification is the term for this process. The saponification value of a fat sample can be estimated using the amount of potassium hydroxide used during hydrolysis. The saponification value is the number of milligrams of potassium hydroxide (KOH) necessary to saponify one gramme of fat.
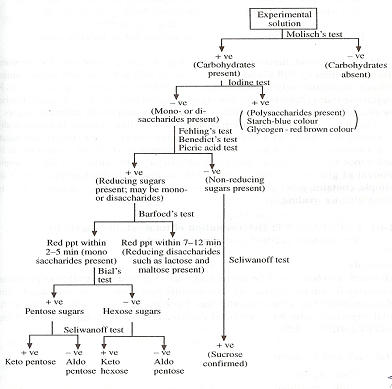
In the above procedure, 3 molecules of KOH are consumed for saponification of each molecule of triacylglycerol, regardless of the fatty acid chain length. Each g of triacylglycerol with shorter chain fatty acids will obviously contain a greater number of triacylglycerol molecules, necessitating a greater amount of KOH. The average molecular weight of the fatty acids in an aclyglyceride is thus determined by the saponification value.
The technique involves refluxing a predetermined amount of alcoholic KOH with a predetermined amount of fat or oil. Back titrating with standardised 0.5N HCl determines the amount of KOH left after hydrolysis, allowing the amount of KOH used for saponification to be quantified.
Materials and Reagents
1. Reflux condenser
2. A bath of boiling water
3.Burette
4. Compounds for testing (tristearin, coconut, corn oil, butter)
5. Fat solvent: a 1:1 v/v mixture of 95% ethanol and ether.
6. 0.5N alcoholic KOH: Prepared a 0.5N KOH solution by dissolving 28.05 g of KOH pellets in 20 ml water and diluting with 95 percent ethanol to 1 L.
7. In a 95 percent alcohol solution, 7.1% phenolphthalein is present.
8. HCl (0.5 N)
Procedure
1. In a conical flask, weigh 1g of fat and dissolve it in around 3 ml of fat solvent (Reagent No. 5).
2. Add 25 mL 0.5N alcoholic KOH, attach a reflux condenser, and reflux the contents for 30 minutes on a boiling water bath.
3. Bring the flask to room temperature and add a few drops of phenolphthalein.
4. Titrate the flask contents with 0.5N HCl until the pink colour fades.
5. Reflux 25 ml of 0.5N alcoholic KOH without any fat sample to create a blank.
Calculations
0.5 N KOH in blank = x ml
0.5 N KOH in test sample = y ml
Titre value for sample = (x – y) ml
28.05 x titre value
Saponification value = ______________________________________
Weight of sample (g)
The multiplication factor of 28.05 in the above equation is included since 1 ml of 0.5N KOH contains 28.05 mg of KOH.
Precautions
1. Because alcohol is highly flammable, an electric heater should be utilised throughout the refluxing process.
2. Effective cooling of the condenser is essential during refluxing to prevent alcohol from evaporating during saponification.
3.5.1 Determination of acid value
The acid value is the amount of potassium hydroxide needed to neutralise one gramme of the substance's free acid.
Recommended procedure
In a 250-mL flask, accurately weigh roughly 10 g of the substance, or the amount stated in the monograph, and add 50 mL of a combination of equal volumes of ethanol (750 g/l) TS and ether R, neutralised with potassium hydroxide (0.1 mol/l) VS following the addition of 1 mL of phenolphthalein/ethanol TS. If necessary, heat until the item is completely dissolved, then cool; titrate with potassium hydroxide (0.1 mol/l) VS, constantly shaking the contents of the flask until a pink colour is obtained that lasts 15 seconds. Take note of the needed mL. (a). Calculate the acid value using the formula below:

3.5.2 Foods- Acid Value
The acid value (AV) is a commonly used parameter in fat and oil specifications. It is a measure of the free fatty acids (FFA) contained in the fat or oil and is defined as the weight of KOH in mg required to neutralise the organic acids present in 1g of fat. Hydrolysis of triglycerides is indicated by an increase in the level of FFA in a sample of oil or fat (Structure on the left). The action of the lipase enzyme causes this reaction, which is a sign of poor processing and storage conditions (i.e., high temperature and relative humidity, tissue damage). The enzyme could be a contamination from other cells, such as bacteria, or it could come from the tissue from which the oil or fat was taken. Hydrolysis of triglycerides produces glycerol in addition to FFA. The acid value of several common oils and bee's wax is shown in the table below.
Oil | Acid Value (AV) |
Canola | 0.071 |
Maize | 0.223 |
Soya | 0.60 |
Virgin olive oil | 6.6 |
Used frying oil | 31 |
Bee's wax | 17-36 |
Flavors and smells are produced by FFA. On the one hand, we have short chain FFA, which are water soluble and volatile with a distinct odour. We have long chain saturated and unsaturated fatty acids on the other hand. In their free state, the latter are more prone to oxidation, and their breakdown products (aldehydes, ketones, alcohols, and organic acids) impart distinct smells and fragrances. These smells and odours are usually regarded as a flaw in oils, fats, and meals that include them. However, there are times when triglyceride hydrolysis and FFA oxidation are necessary for the creation of good flavour and aroma in foods. This is the situation with some processed meats and aged cheeses.
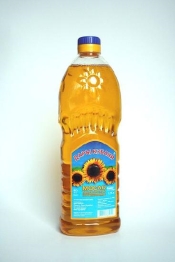
Sunflower seed oil
The AOAC method for determining AV in fats and oils relies on an ethanol titration with phenolphthalein as an indicator. The use of organic solvents (volume and toxicity), the need to heat the reaction media, the oil/incomplete fat's solubility, the need to pre-neutralize the solvents, the use of large amounts of sample, and the possibility of error in detecting the colour change of the indicator when analysing coloured samples are all disadvantages of this and similar methods. Non-titration solutions have been developed to circumvent these drawbacks. 1st Despite its disadvantages, the titration approach is still the most used because it does not necessitate expensive equipment.
3.6.1 Iodine value:
In analytical chemistry, the iodine value, also known as the iodine number, is a measure of the degree of unsaturation of an oil, fat, or wax; it is the quantity of iodine, in grammes, taken up by 100 grammes of the oil, fat, or wax. Iodine is not taken up by saturated oils, fats, or waxes, therefore its iodine value is 0; nevertheless, iodine is taken up by unsaturated oils, fats, and waxes. (Unsaturated compounds have molecules with double or triple bonds, which are iodine reactive.) The more iodine is connected, the greater the iodine value, and the oil, fat, or wax becomes more reactive, less stable, softer, and more vulnerable to oxidation and rancidification. The test involves allowing a known excess of iodine, usually in the form of iodine monochloride, to react with a given weight of oil, fat, or wax, and then titrating the amount of iodine that remains unreacted.
The iodine content of drying oils used in the paint and varnish industries is relatively high (about 190). Semidrying oils, such as soybean oil, have iodine levels that are in the middle (about 130). Iodine levels in non-drying oils, such as olive oil, used in soapmaking and culinary applications, are low (about 80).
3.6.2 Determination of iodine value:
The weight of halogens expressed as iodine absorbed by 100 parts by weight of the substance is the iodine value of that substance. The amount of material used in the test should be sufficient to ensure that at least 70% of the iodine administered in the suggested protocol is not absorbed. Depending on the expected iodine value, the quantity of the material listed in the following table should be used for the determination unless otherwise specified in the monograph:
Iodine value | Quantity of substance in g |
Less than 20 | 1.0 |
20-60 | 0.5-0.25 |
60-100 | 0.25-0.15 |
More than 100 | 0.15-0.10 |
Recommended procedure
In a dry 300-mL to 500-mL stoppered flask, place an amount of the test substance, properly weighed, as described in the monograph, add 15 mL of carbon tetrachloride R, and dissolve. Unless otherwise specified in the monograph, add 25 mL of iodine bromide TS, insert the stopper, previously moistened with potassium iodide (80 g/l) TS, gently shake the flask, and keep in the dark for 30 minutes. Add 20 mL potassium iodide (80 g/l) TS and 150 mL water to a flask and titrate with sodium thiosulfate (0.1 mol/l) VS while shaking the contents of the flask, using starch TS as an indicator at the conclusion of the titration. Take note of the needed mL. (a). Simultaneously, perform the operation in the same way but without the chemical being tested, and record the number of mL of sodium thiosulfate (0.1 mol/l) VS necessary (b). Calculate the iodine value using the formula below.

3.7.1 Flavour Reversion in Fats and Oils:
Flavour Reversion is a modification in edible fats characterised by the formation of an undesirable flavour in the refined material prior to the beginning of real rancidity. It can happen when the fat is exposed to ultraviolet or visible light, or when the fat is heated. The reaction, which is catalysed by the presence of small amounts of metals such as iron and copper, appears to require a small amount of oxygen. Selective hydrogenation reduces linolenic acid levels and helps to prevent flavour reversion. Flavor reversion is most common in soyabean oil.
Factors that influence flavour reversion-
a) Metals like iron, copper
b) Presence of U-V rays
c) Heat
d) Oxygen
Flavor reversion is more prominent in some fats like-Soybean oil has a tendency to have ‘Beany’, ‘haylike’ and eventually ‘fishy’ off flavours.
Prevention- the addition of sequester ants such as-
Ethylene dioxide tetraacetic acid (EDTA)
3.7.2 Rancidity of Food: Introduction, Types, Factors and Prevention of Rancidity | Food Chemistry | Biotechnology
Introduction to Rancidity:
The term "rancidity" refers to the process of food containing fat and oil coming into contact with ambient oxygen and undergoing auto-oxidation, which results in a foul odour and a change in taste. Almost any meal has the potential to go rotten. The word is especially applicable to oils. Oils are especially vulnerable to rancidity due to their chemistry, which makes them vulnerable to oxygen attack.
A metabolic interaction between fats and oxygen causes oxidation of fats. Long-chain fatty acids are destroyed and short-chain molecules are generated during this process. Butyric acid is one of the reaction products, and it is this acid that gives the rotten taste. The degradation of fats, oils, and other lipids by hydrolysis, oxidation, or both is known as rancidification. In glycerides, hydrolysis separates fatty acid chains from the glycerol backbone.
These free fatty acids can subsequently be auto-oxidized further. Unsaturated fats are oxidised largely through a free radical-mediated mechanism. In rancid foods and oils, these chemical processes can produce highly reactive molecules, which are responsible for the unpleasant and toxic aromas and flavours. Food nutrients may be destroyed as a result of these chemical reactions. Vitamins in food can be destroyed by rancidity in some circumstances.
Oil Reversion:
Unsaturated fatty acids are the most essential components in oils like soyabean oil. The triglyceride content of soyabean oil is high in monounsaturated (23 percent oleic acid) and polyunsaturated fatty acids (57 percent linoleic acid; 7 percent linolenic acid). Linolenic acid comprises three unsaturated bonds that are rapidly oxidised. Oil undergoes oxidative deterioration due to the high amount of linolenic and linolenic acid, resulting in colour change and a fishy or painty odour. Oil reversion is the term for this process.
Mechanism of Oil Reversion:
The fundamental mechanism involved in oil reversion is oxidation. Linolenic acid is ten times more sensitive to oxidation than linoleic acid and one hundred times more sensitive than oleic acid. Double bond oxidation is a radical-driven reaction.
Radical reactions typically have three steps:
1. An energy source (heat; light) creates a radical on the fatty acid in the initiating process.
2. An oxygen propagation phase produces peroxides, which react with additional unsaturated fatty acids to produce new radicals.
3. An interaction between two radicals that results in the formation of a new single bond.
3.7.3 Types of Rancidity:
There are two types of rancidity:
1. Oxidative Rancidity:
“Oxidative rancidity” refers to rancidity that occurs as a result of oxygen degradation to foods. During the process, oxygen molecules interact with the oil's natural structure, altering its odour, taste, and safety for consumption, i.e., fat is oxidised and decomposes into compounds with shorter carbon chains, such as fatty acids, aldehydes, and ketones, all of which are volatile and contribute to the rancid fat's unpleasant odour. The development of both unpleasant and dangerous chemicals is caused by oxidative rancidity.
Three distinct classes of substance occurring in oxidized fat have been shown to be toxic:
I Fatty acids that have been peroxidized (peroxidised fatty acids destroy both vitamin A and E in foods).
Polymeric substance
(ii) (under normal food processing conditions these appear in small enough quantities to be insignificant).
Oxidized sterols
(iii) (thought to be involved in the causation of atherosclerotic disease).
2. Hydrolytic Rancidity:
Enzymatic hydrolysis of fats results in the release of free fatty acids from glycerides, resulting in a rotten odour. This is known as hydroiytic rancidity. In glycerides, hydrolysis separates fatty acid chains from the glycerol backbone. Further auto-oxidation of these free fatty acids results in oxidative rancidity.
3.7.4 Factors Affecting Rancidity and Reversion:
1. Oxidation:
Because lipids are eight times more soluble in oxygen than water, the oxidation that results from this exposure is the primary source of rancidity. Unsaturated fats are oxidised largely through a free radical-mediated mechanism. In rancid foods and oils, these chemical processes can produce highly reactive molecules, which are responsible for the unpleasant and toxic aromas and flavours. Auto-oxidation, often known as oxidative rancidity, is the name given to this process.
2. Hydrolysis:
Under the right conditions, triglycerides react with water to generate diglycerides and free fatty acid residues. Monoglycerides and fatty acids are formed when diglycerides mix with water. Finally, the monoglycerides hydrolyzed entirely, yielding glycerol and fatty acids. This is known as hydrolytic rancidity.
3. Presence of Microorganisms – Microbial Lipase:
Lipase is a hydrolytic enzyme produced by certain bacteria that directly interferes with the breakdown of triglycerides to create glycerols and fatty acids. These fatty acids become rancid due to auto-oxidation. For its activity on fats and oils, microbial lipase requires the right pH and other conditions.
4. Presence of Unsaturation in Fatty Acid Chain:
When unsaturated components of a fatty material are exposed to air, they are transformed to hydroperoxides, which then break down into volatile aldehydes, esters, alcohols, ketones, and hydrocarbons, some of which have unpleasant odours. The aforementioned process, as well as hydrolysis, which releases volatile and malodorous acids, mainly butyric acid, causes butter to get rancid. Saturated fats, such as beef tallow, resist oxidation and turn rancid at room temperature.
5. Polyunsaturation:
The higher a fat's polyunsaturation, the faster it will go rancid. Animal fats must become several times more rancid than vegetable oils. Oils and fats with polyunsaturated fatty acids are more prone to rancidity than monosaturated and other forms of saturated fatty acids.
6. Chemical Structure of Oils and Fats:
Oils and fats that are chemically more complex and include a greater number of double bonds, carboxyl or hydroxyl groups have a greater likelihood of becoming rancid. Auto-oxidation is aided by the double bonds found in fats and oils. Auto-oxidation is particularly common in oils with a high degree of unsaturation.
Determining the peroxide value is the best test for auto-oxidation (oxidative rancidity). In the auto-oxidation reaction, peroxides are intermediates. The peroxide value of an oil or fat is used to determine how far rancidity reactions have progressed during storage.
7. Temperature and pH:
These are the major factors that cause fat- and oil-rich foods to get rancid. The hydrolytic action of microbial lipase requires a specific temperature and alkaline pH. The auto-oxidation and hydrolysis are influenced by temperature and pH in an indirect manner.
8. Heat and Light:
Heat and light speed up the rate at which lipids react with oxygen, i.e., heat speeds up auto-oxidation. The formation of free radicals in rancidity and reversion of oils and fats is fueled by heat and light.
3.7.5 Prevention of Rancidity:
Rancidity can be prevented by several ways which are mentioned briefly:
1. Addition of Antioxidants:
Antioxidants are the most effective way to keep food from becoming rancid. Antioxidants are added to fat containing foods in order to retard the development of rancidity due to oxidation.
There are five types of antioxidants:
(1) Natural antioxidants.
(2) Synthetic antioxidants.
(3) Semi-synthetic antioxidants – gallic acid, propylgallate.
(4) Metal chelators – citric acid, phosphoric acid.
(5) Oxygen scavengers – ascorbic acid.
Flavonoids, polyphenols, ascorbic acid (vitamin C), and tocopherols are all natural antioxidants (vitamin E). Butylated hydroxyanisole (BHA), butylated hydroxytoluene (BHT), propyl-3, 4, 5-trihydroxybenzoate (also known as propyl gallate), and ethoxyquin are examples of synthetic antioxidants. Natural antioxidants have a short shelf life, whereas synthetic antioxidants have a longer shelf life and perform better.
Water-soluble antioxidants are ineffective in stopping direct oxidation within fats, but they are useful in intercepting free radicals that pass via food's watery portions. A mix of water-soluble and fat-soluble antioxidants, usually in a fat-to-water ratio, is excellent.
2. Addition of Sequestering Agents:
Metals are bound by sequestering agents, which prevent them from initiating auto-oxidation. EDTA (ethylene diamine tetra acetic acid) and citric acid are examples of sequestering agents.
3. Proper Storage of Fats and Oil Food:
Another strategy to prevent food from becoming rancid is to store it properly, away from the effects of oxygen. Because heat and light accelerate the rate of reactivity of lipids with oxygen, rancidification can be reduced by storing fats and oils in a cold, dark environment with little exposure to oxygen or free radicals.
Do not add new oil to vessels that already have old oil in them. The old oil will cause a reaction, causing the new oil to get rancid more faster than if it were stored in a clean, empty vessel. Allow tanks to drain and dry thoroughly before use, as this will speed up the problems related with oxidation.
3.8.1 Energy and Metabolism
The concept of energy flow ([link]) through living systems, such as cells, is referred to as bioenergetics by scientists. Stepwise chemical reactions are used in cellular processes such as the construction and dismantling of complex compounds. Some of these chemical processes occur naturally and release energy, while others necessitate the use of energy. Cells must constantly produce additional energy to replace that used by the many energy-demanding chemical reactions that occur all the time, just as living beings must consume food to replenish their energy resources. The cell's metabolism refers to all of the chemical reactions that take place inside it, including those that use or generate energy.
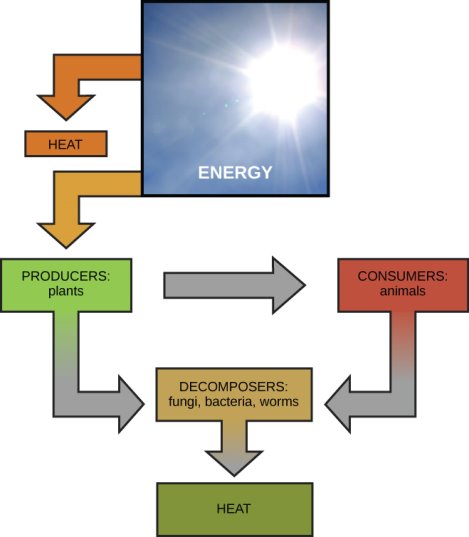
Most life forms acquire their energy from the sun in the end. Herbivores eat plants to obtain energy, and plants employ photosynthesis to gather sunlight. Herbivores are eaten by carnivores, and breakdown of plant and animal matter adds to the nutrient pool.
3.8.2 Metabolic Pathways
Consider the sugar metabolism. This is a famous illustration of one of the many energy-using and energy-producing cellular processes. Sugars are an important source of energy for living things because sugar molecules have a lot of energy trapped within their bonds. These sugars are mostly produced by photosynthesizing organisms like plants. Plants employ energy (derived from sunshine) to transform carbon dioxide gas (CO2) into sugar molecules during photosynthesis (like glucose: C6H12O6). As a waste product, they consume carbon dioxide and create oxygen. This reaction can be summarised as follows:

This process necessitates energy input since it includes the creation of an energy-storing molecule. Energy is provided by a molecule called adenosine triphosphate (ATP), which is the primary energy currency of all organisms, during the light reactions of photosynthesis. Cells use ATP molecules as energy money to accomplish immediate work, similar to how the dollar is used to buy goods. Energy-storing molecules, such as glucose, on the other hand, are consumed only to be broken down and used for energy. The reverse reaction to photosynthesis is a reaction that harvests the energy of a sugar molecule in cells that require oxygen to thrive. This process consumes oxygen while emitting carbon dioxide as a waste product. The following is a summary of the reaction:

Both of these reactions are multi-step processes.
Two metabolic pathways are illustrated by the processes of creating and breaking down sugar molecules. A metabolic pathway is a chain of chemical processes that begins with a starting molecule and gradually alters it through a number of metabolic intermediates, eventually giving a final product. The first metabolic process produced sugar from smaller molecules, while the second process broke sugar down into smaller molecules in the case of sugar metabolism. Anabolic pathways (creating polymers) and catabolic pathways (breaking down polymers into their monomers) are the two opposite processes, with the first consuming energy and the second producing it. As a result, metabolism is divided into two parts: synthesis (anabolism) and decay (catabolism) ([link]).
It's crucial to understand that metabolic pathway chemical processes don't happen on their own. A protein called an enzyme facilitates or catalyses each chemical step. Enzymes are required for catalysing all biological reactions, including those that need and those that release energy.
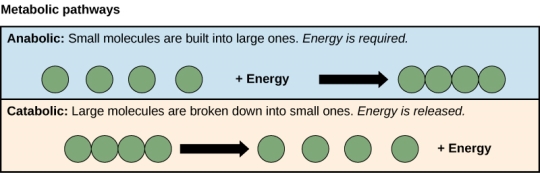
Catabolic pathways are energy-generating routes that break down bigger molecules. Energy is required for anabolic pathways to produce bigger molecules. Maintaining the cell's energy balance necessitates both sorts of pathways.
3.8.3 Energy
The study of energy and energy transfer in physical matter is known as thermodynamics. A system is the matter that is relevant to a specific case of energy transfer, and the surrounds are everything outside of that matter. When heating a pot of water on the stove, for example, the system consists of the stove, the pot, and the water. Within the system, energy is transported (between the stove, pot, and water). Open and closed systems are the two sorts of systems. Energy can be exchanged with the environment in an open system. Because heat can escape through the air, the cooktop system is open. A closed system is incapable of exchanging energy with its environment.
Open systems are biological entities. They exchange energy with their surroundings by using solar energy to perform photosynthesis or consuming energy-storing molecules, and releasing energy to the environment by working and producing heat. Energy, like everything else in the physical universe, is governed by physical laws. The laws of thermodynamics govern the flow of energy inside and between all of the universe's systems.
Energy is described as the ability to conduct labour or bring about change in general. Different types of energy exist. Electric energy, light energy, and heat energy, for example, are all various types of energy. Understanding two of the physical laws that regulate energy is necessary to understanding how energy flows into and out of biological systems.
3.8.4 Thermodynamics
The first law of thermodynamics asserts that the universe's total energy is constant and conserved. To put it another way, the universe has always had and always will have the same quantity of energy. There are many distinct types of energy. Energy can be transported from one location to another or converted into new forms, but it cannot be generated or destroyed, according to the first law of thermodynamics. Every day, energy transfers and transformations take occurring all around us. Electrical energy is converted into light and heat energy by light bulbs. Chemical energy from natural gas is converted into heat energy by gas stoves. Plants convert the energy of sunlight into chemical energy contained within organic molecules, which is one of the most biologically useful energy conversions on the planet ([link]). [link] shows several examples of energy conversions.
All living entities face the difficulty of obtaining energy from their surroundings in forms that may be transferred or transformed into usable energy for labour. To tackle this difficulty, living cells have evolved. Chemical energy held in organic molecules like carbohydrates and lipids is transferred and turned into energy within ATP molecules through a series of cellular chemical processes. The energy stored in ATP molecules is readily available for work. Building complex chemicals, transferring materials, powering the action of cilia or flagella, and contracting muscle fibres to cause movement are all examples of the types of work that cells must undertake.
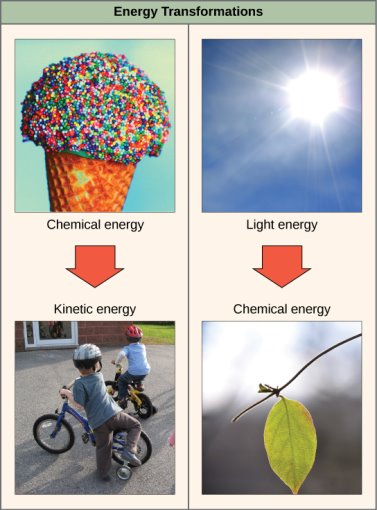
Some examples of energy transfer and transformation from one system to another, as well as from one form to another, are shown. Food gives our cells the energy they need to perform bodily activities, just as light energy gives plants the ability to make the chemical energy they require. (credit "ice cream": D. Sharon Pruitt's work was modified; credit "kids": Max from Providence's work was modified; credit "leaf": Cory Zanker's work was modified)
The fundamental functions of a living cell are to obtain, convert, and use energy to perform labour. The second rule of thermodynamics, on the other hand, explains why these tasks are more difficult than they look. There is no such thing as a 100% efficient energy transfer or transformation. Every energy transfer results in the loss of some energy in an unusable form. Most of the time, this is in the form of heat energy. Heat energy is defined thermodynamically as non-workable energy transferred from one system to another. When a light bulb is turned on, for example, some of the electrical energy transformed into light energy is wasted as heat energy. Similarly, during cellular metabolic activities, some energy is lost as heat energy.
The concept of order and disorder is crucial in physical systems. The more energy a system loses to its environment, the less ordered and unpredictable the system becomes. Entropy is a term used by scientists to describe the measure of randomness or disorder within a system. The term "high entropy" refers to a state of high chaos and low energy. The entropy of molecules and chemical reactions varies as well. Entropy increases, for example, as molecules in a high concentration disperse and spread out. Energy will always be lost as heat in energy exchanges or transformations, according to the second rule of thermodynamics.
Living things are highly organised, and maintaining a low entropy condition necessitates constant energy input.
3.8.5 Potential and Kinetic Energy
There is energy connected with a thing when it is in motion. Consider a wrecking ball. Even a slow-moving wrecking ball can cause significant harm to nearby items. Kinetic energy is the energy associated with moving objects. Kinetic energy can be found in a speeding bullet, a walking person, and the rapid movement of molecules in the air (which produces heat).
What if a crane lifts the identical unmoving wrecking ball two stories above ground? Is there energy involved with the suspended wrecking ball if it isn't moving? Yes, it is correct. The energy used to elevate the wrecking ball did not vanish; instead, it is now stored in the wrecking ball due to its position and the gravitational force acting on it. Potential energy is the name for this form of energy. If the ball were to fall, the potential energy would be converted to kinetic energy until the ball's potential energy was depleted when it hit the ground. Wrecking balls likewise swing like a pendulum; there is a continual transition of potential energy (greatest at the top of the swing) to kinetic energy throughout the swing (highest at the bottom of the swing). The energy of water stored behind a dam or a person ready to parachute out of an aeroplane are two other instances of potential energy.
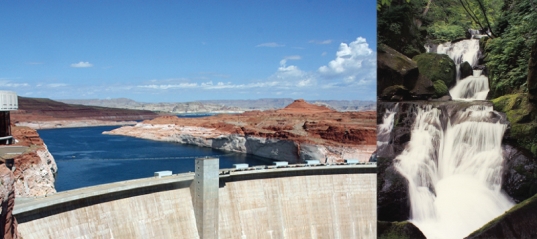
Moving water, such as a cascade or a fast-flowing river, has kinetic energy while still water contains potential energy. (credit "dam": "Pascal"/Flickr modified the work; credit "waterfall": Frank Gualtieri modified the work)
Potential energy is linked not just to the location of matter, but also to the structure of matter. When a spring is compressed, it possesses potential energy, just like when a rubber band is pulled taut. On a molecular level, the bonds that keep molecules' atoms together form a specific structure with potential energy. Keep in mind that anabolic cellular processes use energy to build complex molecules from simpler ones, while catabolic pathways use energy to break down complex compounds. The fact that the breaking of some chemical bonds can release energy means that those bonds contain potential energy. In fact, the bonds of all the food molecules we ingest contain potential energy that is eventually harnessed for use. This is due to the fact that when these bonds are disrupted, they might release energy. Chemical energy is the form of potential energy that resides within chemical bonds and is released when those connections are broken. Chemical energy is responsible for supplying energy to living cells from food. When the chemical links between food molecules are broken, energy is released.
3.8.6 Free and Activation Energy
Following the discovery that chemical reactions release energy when energy-storing bonds are broken, the following is an essential question to consider: What are the methods for calculating and expressing the energy involved with these chemical reactions? How can one reaction's energy be compared to the energy generated by another? These energy exchanges are quantified using a free energy measurement. Remember that all energy transfers, according to the second law of thermodynamics, result in the loss of some quantity of energy in an unusable form, such as heat. The energy associated with a chemical reaction that remains after losses are taken into account is referred to as free energy. In other terms, useful energy is energy that can be used to perform work.
When energy is released during a chemical process, the change in free energy, denoted by the letter G (delta G), is negative. Because the reactants release some free energy during the reaction, a negative change in free energy also signifies that the reaction products have less free energy than the reactants. Exergonic reactions are reactions that have a negative change in free energy and hence release free energy. Exergonic implies that energy is leaving the system. These reactions are also known as spontaneous reactions, and the products have less energy stored in them than the reactants. A difference must be made between the term "spontaneous" and the concept of a chemical reaction occurring instantly. A spontaneous reaction, contrary to popular belief, is not one that occurs suddenly or fast. The rusting of iron is an example of a spontaneous reaction that happens gradually over time.
If a chemical reaction absorbs energy instead of releasing it on balance, the G value for that reaction will be positive. The products have more free energy than the reactants in this scenario. As a result, the reactions' products might be regarded of as energy-storing molecules. These non-spontaneous chemical reactions are known as endergonic reactions. Without the addition of free energy, an endergonic reaction will not take place.
Regarding endergonic and exergonic reactions, there is another crucial aspect to consider. Before they may proceed with their energy-releasing phases, exergonic reactions require a little amount of energy input to get started. Although these reactions yield a net energy release, they nevertheless require some energy input at the start. The activation energy is the little amount of energy required for all chemical reactions to take place.
3.8.7 Enzymes
A catalyst is a substance that aids the occurrence of a chemical reaction, and enzymes are the molecules that catalyse biochemical events. The majority of enzymes are proteins that play an important role in decreasing the activation energy of chemical processes within the cell. At normal temperatures, most of the reactions critical to a live cell happen too slowly to be of much service to the cell. Life could not exist without enzymes to speed up these reactions. Enzymes accomplish this by attaching to the reactant molecules and keeping them in a position that facilitates the chemical bond-breaking and formation processes. It's vital to remember that whether a reaction is exergonic (spontaneous) or endergonic is unaffected by enzymes. This is due to the fact that they have no effect on the free energy of the reactants or products. They just lower the activation energy necessary to proceed with the reaction. Furthermore, the reaction that an enzyme catalyses has no effect on the enzyme itself. After catalysing one reaction, the enzyme is free to participate in subsequent processes.
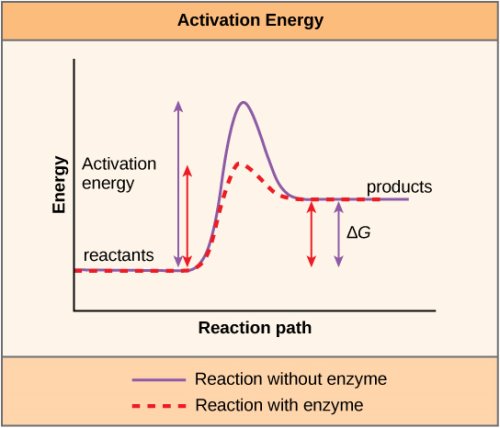
Enzymes reduce the reaction's activation energy while leaving the reaction's free energy unchanged.
The substrates of an enzyme are the chemical reactants to which it binds. Depending on the chemical reaction, there may be one or several substrates. A single reactant substrate can be broken down into several products in some reactions. In some cases, two substrates may combine to form a single bigger molecule. Two reactants may enter a process and both become changed, yet they exit as two different products. The enzyme's active site is the place within the enzyme where the substrate binds. The “action” takes place on the active site. Because enzymes are proteins, the active region has a unique mix of amino acid side chains. Each side chain has its own set of characteristics. Large or little, weakly acidic or basic, hydrophilic or hydrophobic, positively or negatively charged, or neutral, they come in many shapes and sizes. Within the active site, the particular mix of side chains generates a very particular chemical environment. This milieu is ideal for binding to a single chemical substrate (or substrates).
The local environment has an influence on active sites. Increasing the temperature of the environment often increases reaction rates, whether enzyme-catalyzed or not. Temperatures outside of an ideal range, on the other hand, slow down the pace at which an enzyme catalyses a reaction. Enzymes denature at high temperatures, resulting in an irreversible alteration in their three-dimensional form and, as a result, their function. Enzymes are also best adapted to function within a specific pH and salt concentration range, and extreme pH and salt concentrations, like temperature, can cause enzymes to denature.
For a long time, scientists believed that enzyme-substrate interaction was a straightforward “lock and key” process. This paradigm claimed that the enzyme and substrate were fully compatible in a single instantaneous step. Current research, on the other hand, supports a paradigm known as induced fit. The induced-fit model extends the lock-and-key paradigm by explaining a more dynamic enzyme-substrate binding. As the enzyme and substrate contact, a minor alteration in the enzyme's structure occurs, resulting in a perfect binding configuration between the enzyme and substrate.
An enzyme-substrate complex is generated when an enzyme binds to its substrate. This compound decreases the reaction's activation energy and supports its rapid development in one of several ways. On a basic level, enzymes aid chemical reactions involving several substrates by bringing them together in the most favourable reaction orientation. Another method enzymes stimulate substrate reaction is by generating an ideal environment for the reaction to take place within the active site. The chemical characteristics that result from the exact arrangement of amino acid R groups within an active site provide the ideal environment for the reaction of an enzyme's unique substrates.
By weakening the bond structure and making it easier to break, the enzyme-substrate combination can reduce activation energy. Finally, by participating in the chemical reaction, enzymes can reduce activation energies. It's vital to note in these situations that the enzyme will always revert to its natural condition once the reaction is finished. One of the most distinguishing characteristics of enzymes is that they are unaffected by the reactions they catalyse. After catalysing a reaction, an enzyme releases its product(s) and is ready to catalyse another.
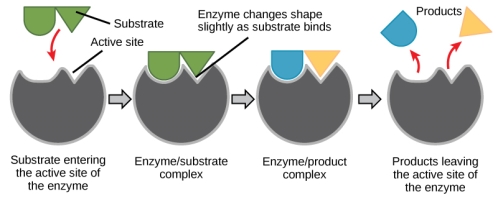
The induced-fit model is a variant of the lock-and-key model that describes how enzymes and substrates change dynamically throughout the transition state to boost substrate affinity for the active site.
It would appear perfect if all of an organism's enzymes were abundant and functioned properly in all biological conditions, in all cells, at all times. However, a number of processes prevent this from happening. Cellular requirements and circumstances vary from cell to cell and alter over time within individual cells. Stomach cells require different enzymes than fat storage cells, skin cells, blood cells, and nerve cells. Furthermore, a digestive organ cell works far more to assimilate and break down nutrients immediately after a meal than it does several hours later. The quantity and functionality of individual enzymes must fluctuate depending on the cellular demands and environment.
Because activation energy controls the speeds of biochemical reactions, and enzymes decrease and determine activation energies for chemical processes, the relative numbers and functions of the various enzymes within a cell ultimately dictate which processes will take place and at what rates. In cells, this determination is closely regulated. Enzyme activity is influenced by environmental parameters such as pH, temperature, salt content, and, in rare circumstances, cofactors or coenzymes in particular cellular settings.
Enzyme activity can also be modulated in ways that promote or inhibit it. There are a variety of substances that inhibit or boost enzyme action, as well as a variety of processes via which they do so. An inhibitor molecule is similar enough to a substrate in some circumstances of enzyme inhibition that it can attach to the active site and merely prevent the substrate from binding. Because an inhibitor molecule competes with the substrate for binding to the active site, the enzyme is inhibited by competitive inhibition.
In non-competitive inhibition, on the other hand, an inhibitor molecule binds to the enzyme in an allosteric region other than the active site, yet nonetheless manages to block substrate binding to the active site. Some inhibitor chemicals bind to enzymes in a way that causes a conformational change in the enzyme, lowering its affinity for its substrate. Allosteric inhibition is the name for this sort of inhibition. The majority of allosterically controlled enzymes are made up of several polypeptides, which means they include several protein subunits. When an allosteric inhibitor binds to a specific location on an enzyme, all active sites on the protein subunits are slightly altered, making them less efficient at binding their substrates. Both allosteric activators and inhibitors exist. Allosteric activators bind to enzymes at sites other than the active site, causing a conformational shift that improves the enzyme's active site(s) affinity for its substrate (s).
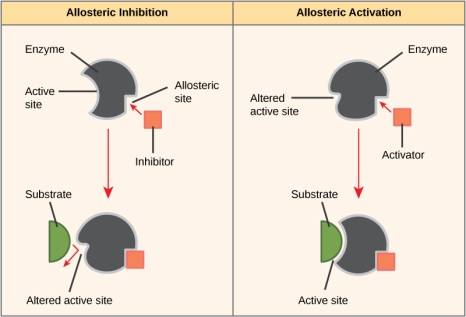
Allosteric inhibition acts by causing an indirect conformational shift in the active site, preventing the substrate from fitting. In allosteric activation, on the other hand, the activator molecule changes the shape of the active site to allow the substrate to fit better.
Key Takeaways:
- Energy is required for all living creatures to develop and reproduce, maintain their structures, and respond to their environments; metabolism is the collection of mechanisms that make energy accessible for cellular functions.
- Metabolism is a collection of chemical reactions that are spontaneous and release energy, as well as chemical reactions that are non-spontaneous and require energy to progress. • In order to carry out cellular operations, living organisms must consume energy from food, nutrients, or sunshine.
- Energy is required for the transport, synthesis, and breakdown of nutrients and molecules in a cell.
3.9.1 Cells obtain energy by the oxidation of organic molecules:
All animal and plant cells are powered by energy stored in the chemical bonds of organic molecules, whether these be sugars that a plant has photosynthesized as food for itself or the mixture of large and small molecules that an animal has eaten. Organisms must extract this energy in a useful form in order to utilise it to live, grow, and reproduce. Energy is taken from food molecules by a process of slow oxidation, or controlled burning, in both plants and animals.
The atmosphere of the Earth contains a lot of oxygen, and in the presence of oxygen, the most energetically stable form of carbon is CO2, while the most energetically stable form of hydrogen is H2O. A cell can obtain energy from sugars or other organic molecules by combining their carbon and hydrogen atoms with oxygen to form CO2 and H2O, respectively, a process known as respiration.
Photosynthesis and respiration are two processes that work together (Fig). Nearly all organisms absorb the oxygen created by photosynthesis in the burning of organic molecules. And some of the CO2 molecules that are fixed today into organic molecules by photosynthesis in a green leaf were yesterday released into the atmosphere by the respiration of an animal or by that of a fungus or bacterium decomposing dead organic matter.
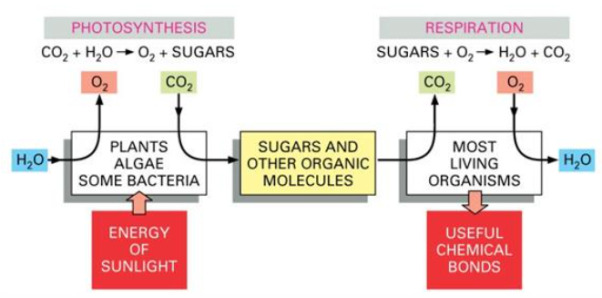
Figure. Photosynthesis and respiration as complementary processes in the living world
As a result, we can see that carbon use is part of a larger cycle that incorporates the biosphere (all living things on Earth) as a whole, as well as individual organisms. Similarly, nitrogen, phosphorus, and sulphur atoms travel between the living and non-living worlds in cycles involving plants, animals, fungi, and bacteria.
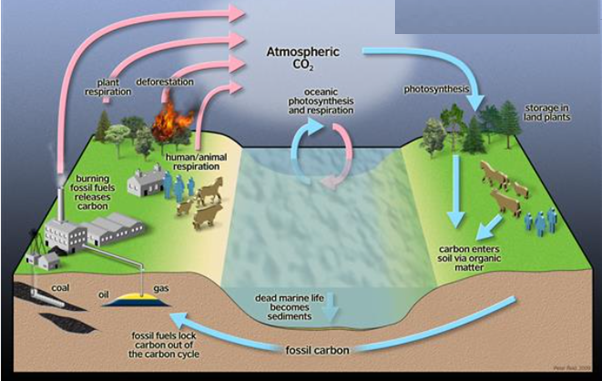
Figure. The carbon cycle
Oxidation and Reduction Involve Electron Transfers
When organic material is burned in a fire, the cell does not oxidise organic molecules in one step. Metabolism passes molecules through a wide variety of processes via enzyme catalysts, with only a few of them involving the direct addition of oxygen. In the context of this definition, oxidation refers to more than just the addition of oxygen atoms. Rather, it refers to any reaction that involves the transfer of electrons from one atom to another. In this context, oxidation refers to the removal of electrons, while reduction refers to the addition of electrons. Oxidation and reduction always happen at the same time, which means that if one molecule receives an electron in a reaction (reduction), another molecule loses an electron (oxidation). When a sugar molecule is oxidised to CO2 and H2O, the O2 molecules involved in the formation of H2O gain electrons and are thus reduced.
When a molecule in a cell accepts an electron (e-), it frequently also accepts a proton (H+) (protons being freely available in water). In this situation, the overall result is to add a hydrogen atom to the molecule:
A + e- + H+ → AH
Despite the fact that a proton and an electron are involved (rather than just one electron), hydrogenation processes are reductions, while dehydrogenation reactions are oxidations. It's very simple to detect if an organic molecule is being oxidised or reduced: reduction occurs when the number of C-H bonds in the molecule grows, whereas oxidation occurs when the number of C-H bonds in the molecule drops.
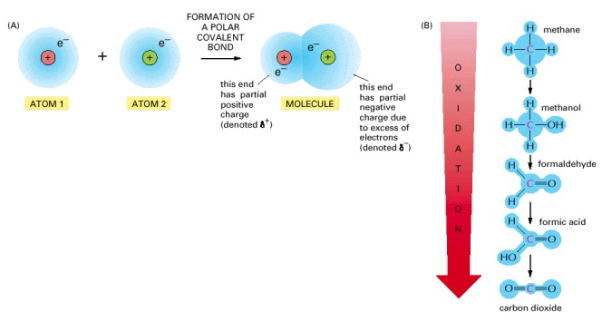
Figure. Oxidation and reduction
3.10.1 Introduction
What's going on inside your body at the moment? Your first response can be that you're hungry, that you're weary, or that your muscles are sore from a run. But let's take it a step further and look at what's going on in your cells, moving past the layer of your consciousness.
If you could look into every cell in your body, you'd find it to be a hive of activity, more akin to a bustling open-air market than a peaceful room. Energy is converted inside your cells, changing forms as molecules conduct the related chemical events that keep you alive and functional, whether you are awake or asleep, exercising or watching TV.
Metabolism is a set of chemical reactions that interconnect in a series of pathways. It is a balancing act between the building and breakdown of molecules in the body.
Type of metabolism | Process | Energetics | Example |
Anabolism | Builds complex molecules from simple ones | Endergonic | Production of new body tissues |
Catabolism | Complicated molecules are broken down into simpler ones. | Exergonic | The process of food digestion |
Small molecules are assembled into larger molecules in the anabolic process. Typically, energy is necessary.
Large compounds are broken down into smaller molecules via the catabolic process. Normally, energy is released.
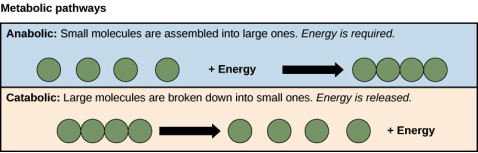
Anabolic vs catabolic pathways.
3.10.2 Anabolic and catabolic
1. Catabolism Definition
Catabolism refers to a group of biological reactions that break down large compounds into smaller ones. Cells use catabolic processes to create energy or fuel anabolism because they are thermodynamically advantageous and spontaneous. Catabolism is an exergonic process that produces heat through hydrolysis and oxidation.
Cells can store important raw materials in complex molecules, break them down by catabolism, and reuse the smaller molecules to create new products. Protein, lipid, nucleic acid, and polysaccharide catabolism, for example, produces amino acids, fatty acids, nucleotides, and monosaccharides, respectively. Carbon dioxide, urea, ammonia, acetic acid, and lactic acid are some of the waste products that are produced.
Catabolism: Cellular respiration
Cellular respiration is a catabolic process that breaks down glucose into useful energy for a cell.
The entire cellular respiration reaction is:

Cellular respiration, like all catabolic processes, releases energy that can be harnessed and utilised by other reactions in the cell.
2. Anabolism Definition
Anabolism, often known as biosynthesis, is a group of biological operations that build molecules from smaller parts. Anabolic reactions are endergonic, which means they don't happen on their own and require energy input to continue. Anabolic and catabolic reactions are usually linked, with catabolism providing anabolism's activation energy. Many anabolic processes are powered by the breakdown of adenosine triphosphate (ATP). The mechanisms of anabolism are, in general, condensation and reduction processes.
Anabolism: Photosynthesis
Photosynthesis is an anabolic process in which plants transform carbon dioxide gas and water into sugar molecules using energy from the sun.
The entire photosynthetic reaction is as follows:

Photosynthesis, like all anabolic processes, consumes energy as glucose is built. Some of this glucose is consumed by the plant, and some of it is used as food by other species.
Key takeaway:
- Metabolism is made up of two broad groups of biological reactions: anabolism and catabolism.
- The creation of complex compounds from simpler ones is known as anabolism. These chemical reactions necessitate the use of energy.
- Catabolism is the process of breaking down complex molecules into smaller ones. These reactions result in the release of energy.
- Typically, anabolic and catabolic pathways act in tandem, with catabolism providing energy for anabolism.
3.10.3 Difference Between Catabolism and Anabolism
Difference Between Catabolism and Anabolism | |
Catabolism | Anabolism |
Catabolism is the breakdown of large, complex compounds into smaller, more absorbable molecules. | Anabolism is the process of constructing molecules that are essential for the body's proper functioning. |
Catabolism is a metabolic process that releases energy. | Energy is required for anabolic processes. |
Adrenaline, cytokine, glucagon, and cortisol are hormones that are involved in the processes. | Estrogen, testosterone, growth hormones, and insulin are all involved in the process. |
Proteins decompose into amino acids, glycogen decomposes into glucose, and triglycerides decompose into fatty acids are all examples of catabolic processes. | Polypeptides are made from amino acids, glycogen is made from glucose, and triglycerides are made from fatty acids. |
Potential energy is converted to kinetic energy during catabolism. | Kinetic energy is turned into potential energy during anabolism. |
It is necessary for living entities to carry out a variety of tasks. | It's necessary for upkeep, growth, and storage. |
3.11.1 The Catabolism of Fats and Proteins for Energy:
What does the term catabolism signify, first and foremost? When we talked about catabolic and anabolic reactions, we noted that catabolic reactions break molecules apart. Consider a Catastrophe when things are coming apart and breaking apart to remember what catabolic implies. You could also recall cats who rip your stuff to shreds.
The body breaks down largely carbs, some fats, and very little amounts of protein to generate ATP for energy. Carbs are the go-to, favourite food of cells when it comes to producing ATP, but now we'll look at how our cells use fats and proteins for energy. What we'll discover is that they'll all be converted to sugars (acetyl), as seen in the diagram below.
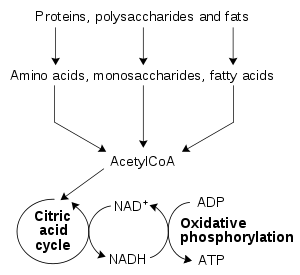
Glycogenesis is the process through which glucose is converted to glycogen. Insulin is the hormone that facilitates this activity. The hormone glucagon then increases glycogenolysis (Glycogen-o-lysis) to break down glycogen and raise blood sugar levels when glycogen has to be broken down.
Phosphoglycerate (PGAL) and then pyruvic acid are formed when glucose is broken down. What do we term the process of pyruvic sugars being separated from glucose? Glycolysis (glyco=glucose, and -lysis means to break down) is what this is. Pyruvic acid is transformed to lactic acid when there isn't enough oxygen. Lactic acid is transformed back to pyruvic acid when oxygen becomes available. Keep in mind that everything happens in the cytoplasm.
The pyruvates are subsequently aerobically broken down into Acetyl-CoA in the mitochondria. The acetyl sugars are broken down completely into carbon dioxide and a quantity of hydrogens are eliminated in the Krebs citric acid cycle. FAD and NAD pick up the hydrogens and shuttle them through a series of coenzymes. Phosphorylation occurs as these hydrogens are transferred, attaching a phosphate group to ADP to generate ATP. Finally, oxidative phosphorylation occurs when hydrogens bind to oxygen.
Let's look at how fats and proteins are processed now.
The Catabolism of Fats
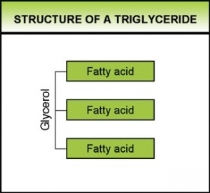
Fats are the next most popular source of energy after sugars. Similarly, to how glucose is stored as glycogen in our liver and muscles, fats are stored as triglycerides in our fat cells. Three saturated fatty acids make up triglycerides. Keep in mind that a fatty acid is nothing more than a long chain of carbons with hydrogens connected. The amount of carbon atoms in fatty acids is always an even amount. They can have 12, 14, 16, 18, 20, 24, or more carbons. There will never be an FA with an odd amount of carbons. This fatty acid is broken down two carbons at a time, resulting in the formation of the two-carbon acetyl sugar. A beta oxidation process is what this is called. Then, as if they were sugars, they are broken down in the Krebs cycle.
A fatty acid, unlike glucose, which has six carbon atoms, is not a tiny molecule. It's more like 24 carbons long, so there would be a whopping 12 acetyl sugars and three fatty acids because it's a triglyceride to begin with. That's incredible! When a triglyceride is catabolized, it produces 36 acetyl sugars at once, flooding the system. Because they can't complete the Krebs cycle quickly enough, some of these acetyl sugars become keto acids. These occur when the body breaks down fats at a faster rate than usual. Fat offers twice as much calories as carbs or protein per gramme. When fat is broken down at a faster pace, more keto acids are produced (aka ketone bodies). It's worth noting that because lipids are converted into acetyl sugars that enter the Krebs cycle, they require oxygen. Sugars are the only foods that can be broken apart without the need for oxygen.
Catabolism of fat –> Formation of ketoacids (“ketone bodies”)
Marathon runners claim that the latter 6 kilometres are more difficult than the first 20. The idiom "struck the wall" is often used. It's as though you can't move. There are a few ideas, but one is that you've used up all of your sugars and have now moved to fats since you need oxygen to make ATP. With sugars, at the very least, you were generating some energy.
The Catabolism of Proteins
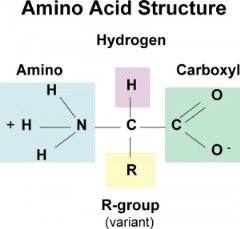
Proteins aren't the most popular source of energy, but the body will use them if it has to. Amino acids make up proteins, so when they're digested, we're left with hundreds or thousands of them.
The image to your right is a representation of an amino acid. It starts with a carbon atom, an amino group on one side, an acid group (COOH) on the other, and a hydrogen on the third. What separates them is what is attached in place of the letter "R."
To utilise amino acids as energy, they must first be converted to sugars. Carbon, hydrogen, and oxygen make up sugars. Carbon and hydrogen make up the majority of fats. Carbon, hydrogen, oxygen, and NITROGEN atoms make up amino acids.
If we want to convert amino acids to sugars, we must first remove the nitrogen. Deamination is the process of eliminating that amino group (taking away the amino group, NH2). When you take away the NH2, you get NH3 (Ammonia). The ammonia is then converted to urea in your liver, which is essentially a carbon and oxygen molecule with two amino groups attached. This urea is released into the bloodstream by your liver and is the most common organic waste carried in our bodies. The amount of urea in your blood is measured clinically and is referred to as the BUN level. The abbreviation BUN stands for Blood Urea Nitrogen (Urea contains Nitrogen). Our kidneys filter this blood, which ultimately appears in our urine as the primary organic waste.
So far, we've discussed how amino groups are removed to eliminate nitrogen, leaving us with carbons, hydrogens, and oxygens, just like a sugar. What is the name of this novel amino acid without the amine group? It's still an acid because it's missing the amino group, and it's known as a keto acid (also known as "ketone bodies"). The ketoacid can be converted to acetyl sugar in a reversible manner.
Amino Acids -> NH2 removed -> NH3 -> Urea is formed in liver and excreted in urine.
There are many types of ketoacids but we’ll mention three of them.
1) Acetone is a very important ketone body.
2) Acetoacetic acid.
3) Beta-hydroxybutyric acid
Acetone has a distinct odour and was once the most often used ingredient in nail polish remover. You can smell acetone on someone's breath when they're creating a lot of ketoacids, much like you can when they've had a lot of alcohol.
Gluconeogenesis is the process through which the body converts sugars that aren't sugars, such as ketoacids into acetyl sugars. This is a term that is usually reserved for proteins.
Gluconeogenesis: Ketoacids -> Acetyl-CoA sugar
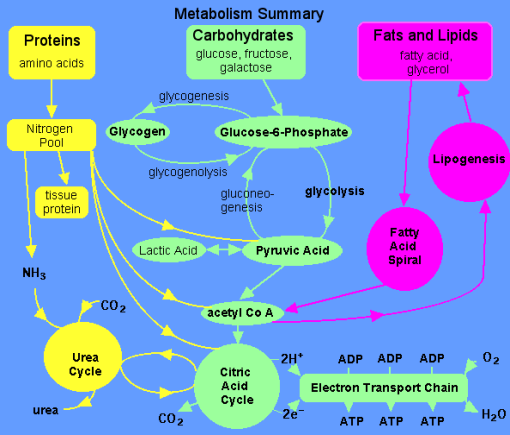
3.11.2 Connections of Carbohydrate, Protein, and Lipid Metabolic Pathways:
Connecting Other Sugars to Glucose Metabolism
To enter the glycolytic route, sugars like galactose, fructose, and glycogen are catabolized into new molecules.
You've learnt about glucose catabolism, which gives living cells their energy. However, living organisms require more than glucose to survive. What happens when a turkey sandwich becomes ATP in your cells? All of the catabolic routes for carbs, proteins, and lipids eventually connect to glycolysis and the citric acid cycle.
Metabolic pathways should be viewed as porous, with chemicals entering from other pathways and intermediates exiting for other routes. These aren't completely closed systems. Many of a pathway's substrates, intermediates, and products are reactants in other routes. Lipid catabolic pathways are linked to glucose catabolic pathways, just as sugars and amino acids.
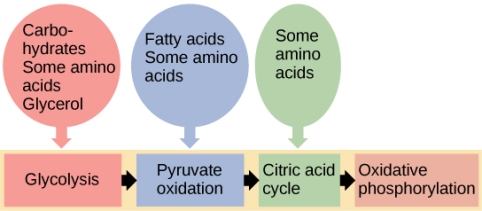
Glycogen Pathway: Glycogen from the liver and muscles, digested into glucose-1-phosphate, can feed into the catabolic pathways for carbohydrates, along with lipids and proteins.
In mammals, glycogen, a polymer of glucose, serves as an energy store molecule. Excess glucose is shifted into glycogen for storage when there is enough ATP. Glycogen is produced and stored in the liver as well as the muscles. If blood sugar levels decline, glycogen is digested into the glucose monomer glucose-1-phosphate (G-1-P). Because glycogen serves as a source of glucose, ATP can be created for a longer time during activity. In both muscle and liver cells, glycogen is broken down into G-1-P and transformed into glucose-6-phosphate (G-6-P), which then enters the glycolytic pathway.
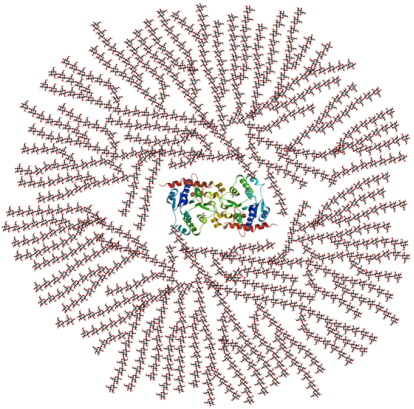
Glycogen Structure: Schematic cross-sectional image of glycogen in two dimensions: Glycogenin has a core protein that is surrounded by glucose unit branches. Around 30,000 glucose units may be found in a single globular granule.
Milk contains galactose, which is a sugar. Lactose is converted to galactose and glucose by an enzyme in the small intestine of infants. Adults have evolved this enzyme in locations where milk products are commonly ingested. Galactose is transformed to G-6-P in the liver and can then be used in the glycolytic pathway.
Fructose, along with glucose and galactose, is one of three dietary monosaccharides that are absorbed directly into the bloodstream following digestion. Fructose is absorbed by the small intestine and then transported to the liver, where it is converted into glycogen. Both fructose and galactose catabolism yield the same number of ATP molecules as glucose catabolism.
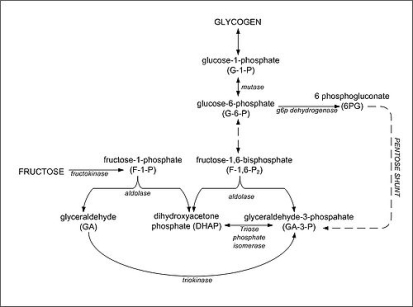
Fructose Metabolism: Although fructose and glucose metabolism share many of the same intermediate components, their metabolic fates in human metabolism are very different.
Sucrose is a disaccharide made up of a glucose molecule and a fructose molecule linked together by a glycosidic connection. Sucrose is broken down into monomers of glucose and fructose during catabolism. Glucose can enter the glycolytic process directly, however fructose must first be converted to glycogen, which can then be broken down to G-1-P and entered the glycolytic route as previously stated.
Connecting Proteins to Glucose Metabolism
Excess amino acids are transformed into compounds that can enter glucose catabolism pathways.
Metabolic pathways should be considered porous, in the sense that substances enter from other pathways and intermediates exit to go to other routes. These aren't completely closed systems. Many of a pathway's substrates, intermediates, and products are reactants in other routes. Proteins are an excellent illustration of this phenomenon. They can be broken down into their individual amino acids and utilised at different stages of the glucose catabolism process.
A range of enzymes in cells hydrolyze proteins. The amino acids are mostly recycled into the production of new proteins or employed as precursors in the production of other vital biological components as hormones, nucleotides, and neurotransmitters. However, if there are too many amino acids or if the body is starving, some amino acids will be diverted to the glucose catabolism pathways.
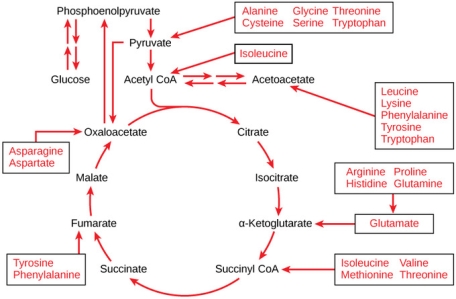
The Relationship Between Amino Acids and Glucose Metabolism Pathways: Proteins provide the carbon skeletons of particular amino acids (shown in boxes), which can feed pyruvate, acetyl CoA, and the citric acid cycle.
Before the carbon chain may enter these routes, each amino acid must have its amino group removed (deamination). The urea cycle converts amino acids that have had their amino group removed into ammonia. The amino acid's remaining atoms form a keto acid, which is a carbon chain with one ketone and one carboxylic acid group. The liver in mammals produces urea by combining two ammonia molecules with one carbon dioxide molecule. As a result, urea is the primary waste product formed in mammals from nitrogen derived from amino acids, and it is excreted in urine. Following that, the keto acid might join the citric acid cycle.
Amino acids can enter the glucose metabolism pathways as pyruvate, acetyl CoA, or numerous components of the citric acid cycle after being deaminated. In the citric acid cycle, deaminated asparagine and aspartate are transformed to oxaloacetate and then into glucose catabolism. Before entering the pathways, deaminated amino acids can be transformed into another intermediary molecule. Multiple amino acids can enter glucose catabolism at different points.
Connecting Lipids to Glucose Metabolism
Parts of the glucose catabolism pathways can both make and break down lipids.
Lipid catabolic pathways are linked to glucose catabolic pathways, just as sugars and amino acids. Cholesterol and triglycerides are lipids that are linked to glucose pathways.
Cholesterol
Cholesterol is a precursor of steroid hormones and contributes to cell membrane flexibility. The synthesis of cholesterol begins with acetyl groups transferred from acetyl CoA and can only go in one direction; it cannot be reversed. As a result, cholesterol production necessitates a glucose metabolism intermediary.
Triglycerides
Glycerol and three fatty acids make up triglycerides, a type of long-term energy storage in animals. Animals can produce the majority of the fatty acids they require. Parts of the glucose catabolism pathways can both make and break down triglycerides. Glycerol can be phosphorylated to glycerol-3-phosphate, which allows glycolysis to continue.
Fatty acids are catabolized in the matrix of the mitochondria by a process termed beta-oxidation, which transforms their fatty acid chains into two carbon units of acetyl groups and produces NADH and FADH2. CoA picks up the acetyl groups and forms acetyl CoA, which then enters the citric acid cycle when it interacts with oxaloacetate. The electron transport chain then uses the NADH and FADH2.
Key takeaway:
- When blood sugar levels fall, glycogen is broken down into glucose -1-phosphate, which is subsequently transformed to glucose-6-phosphate and sent to glycolysis to produce ATP.
- Galactose is converted to glucose-6-phosphate in the liver before entering the glycolytic pathway; fructose is turned to glycogen in the liver before entering glycolysis via the same pathway as glycogen.
- Sucrose is broken down into glucose and fructose, with glucose directly entering the route and fructose being converted to glycogen.
3.12.1 Metabolic Interrelationships:
In the starving and fed cycle, how does this happen?
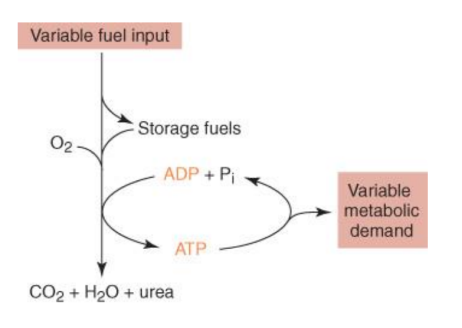
Figure. Humans can use a variable fuel input to meet a variable metabolic demand.
3.12.2 Metabolic Processes:
- Glycogenolysis
- Gluconeogenesis
- Fatty Acid Synthesis
- Lipogenesis
- TCA Cycle Activity
- Amino Acid Oxidation
- Proteolysis
- Glycogenesis
- Glycolysis
- Lipolysis
- Glutaminolysis
- Ketogenesis
- Protein Synthesis
- Urea Synthesis
Glucose raises the ATP levels in B cells
Endothelial lipoprotein lipase hydrolyze Triacylglycerol, and then synthesis
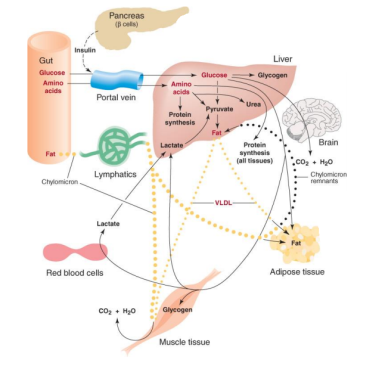
Figure. Disposition of glucose, amino acids, and fat by various tissues in the well-fed state
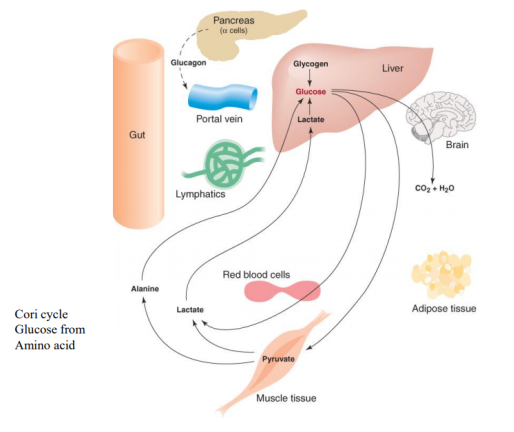
Figure. Metabolic interrelationships of major tissues in early fasting state.
Fatty acids** are not able to be utilised in the production of glucose. Alanine and Glutamine released in large quantities others go to intermediate (puruvate, aKetoglutarate) which also can yield Glutamine and Alanine. Glutamine is used by enterocytes to make pyrimidine and purine. ABy using malic enzyme, ketoglutarate is converted to malate, which is then converted to pyruvate, which is finally converted to alanine. Ketone bodies can decrease muscle wasting by reducing alanine release, proteolysis, and branched AA oxidation.
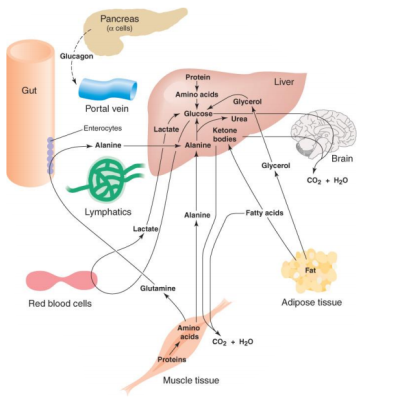
Figure. Metabolic interrelationships of major tissues in fasting state.
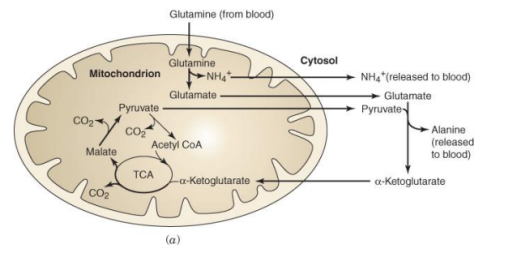
Glutamate is the source of two nitrogen atoms for urea, glutamate oxidative deamination, and aspartate (Ox, Glu).
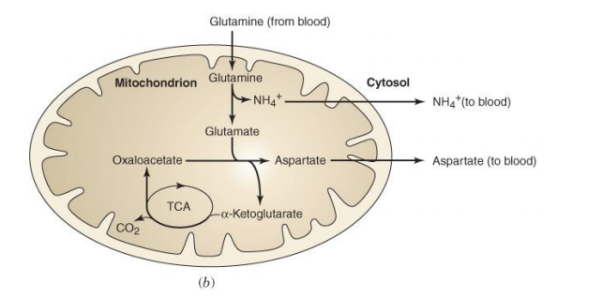
Figure. Glutamine catabolism by rapidly dividing cells
Intestinal epithelium converts glutamine to citrulline, the only tissue that express an ATP dependent glutamate reductase that converts Glu to the Glu semialdehyde that forms Ornithine. In the liver, this prescription is irreversible. This mechanism can be used to control arg and protein breakdown when protein intake is limited. SAM's most significant function in the body (production of Creatine)
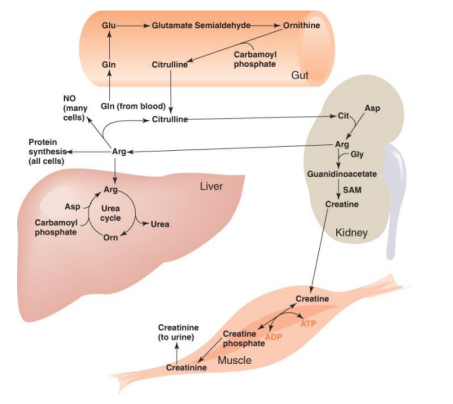
Figure. Gut and kidney function together in synthesis of arginine from glutamine
The liver and kidney are sources of Carnitine for extrahepatic tissue, while lysine residues from protein breakdown are Nmethylated with SAM to create Trimethyllysine TML. Carnitine is produced in the liver and kidneys.
Carnitine is formed when G-butyrobetaine is hydroxylated. Carnitine is not produced by muscle or the heart.
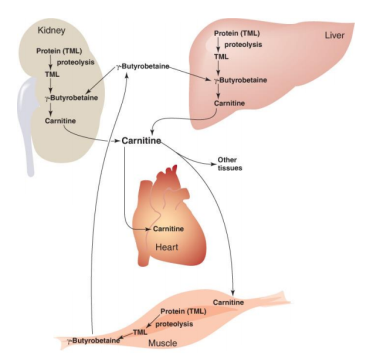
Figure. Kidney and liver provide carnitine for other tissues
Energy needs, reserves, and calorie intake Homeostasis is the state of being in balance.
The average person consumes 180-280 g of carbohydrates, 70-100g of protein and 70-100g of fat daily. This satisfies a daily calorie need of 1600-2400.
Glucose levels must be kept in check.
Table: Energy Reserves of Humans
| Fuel Reserves | ||
Store Fuel | Tissue | (g) | (kcal) |
Glycogen | Liver | 70 | 280 |
Glycogen | Muscle | 120 | 480 |
Glucose | Body fluids | 20 | 80 |
Fat | Adipose | 15000 | 135,000 |
Protein | Muscle | 6000 | 24000 |
Data are for a normal subject weighing 70 kg. Carbohydrate supplies 4 kcal/g; fat 9 kcal/g; protein 4 kcal/g
Table: Substrate and Hormone Levels in Blood or well-fed, fasting and starving humans
Harmone or Substrate | Very well fed | Postabsorptive 12 hours | Fasted 3 days | Starved 5 weeks |
Insulin (U/mL) | 40 | 15 | 8 | 6 |
Glucagon (pg/mL) | 80 | 100 | 150 | 120 |
Insuline glucagon (U/pg) | 0.5 | 0.15 | 0.05 | 0.05 |
Glucose(mM) | 6.1 | 4.8 | 3.8 | 3.6 |
Fatty acids (mM) | 0.14 | 0.6 | 1.2 | 1.4 |
Acetpacetate(mM) | 0.04 | 0.05 | 0.4 | 1.3 |
-Hydroxybutyrate(mM) | 0.03 | 0.10 | 1.4 | 6.0 |
Lactate(mM) | 2.5 | 0.7 | 0.7 | 0.6 |
Pyruvate(mM) | 0.35 | 0.06 | 0.04 | 0.03 |
Alanine(mM) | 0.8 | 0.3 | 0.3 | 0.1 |
ATP equivalents(mM) | 262 | 235 | 301 | 428 |
Phase IV ketone bodies predominate to compensate for fuel needs
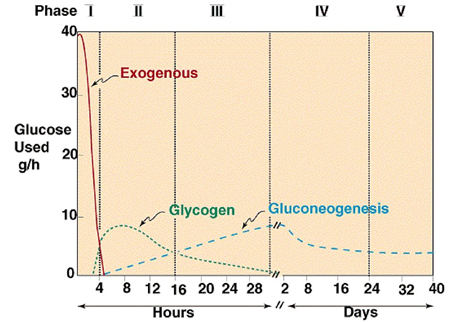
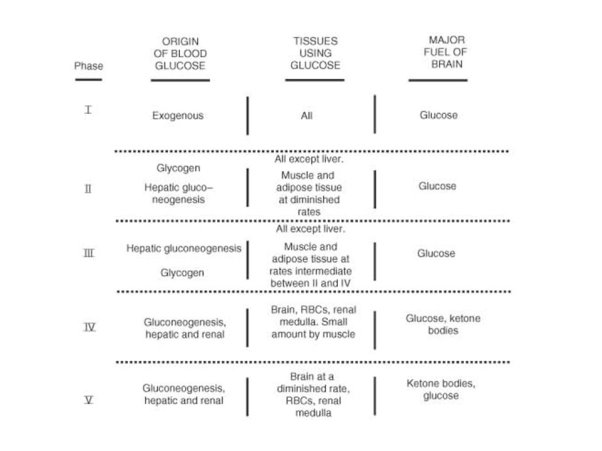
Figure: The five phases of glucose homeostasis
3.13.1 Carbohydrates:
Carbohydrates can be simple or complicated, depending on the size of the molecule.
Simple carbohydrates: Simple carbohydrates include glucose and sucrose (table sugar) in various forms. They are the quickest source of energy because they are small molecules that can be swiftly broken down and absorbed by the body. They cause a rapid rise in blood glucose levels (blood sugar). Simple carbs are abundant in fruits, dairy products, honey, and maple syrup, and they are responsible for the sweetness of most candies and desserts.
Complex carbohydrates: Long sequences of simple carbs make up these carbs. Complex carbohydrates must be broken down into simple carbohydrates before they can be absorbed since they are larger molecules than simple carbs. As a result, they deliver energy to the body more slowly than simple carbohydrates, but faster than protein or fat. They are less likely to be turned to fat because they are digested more slowly than simple carbohydrates. They also raise blood sugar levels more slowly and to lower levels than simple carbs, but for a longer period of time. Starches and fibres, which are found in wheat products (such as breads and pastas), other grains (such as rye and corn), beans, and root vegetables, are examples of complex carbohydrates (such as potatoes and sweet potatoes).
Carbohydrates may be
- Refined
- Unrefined
The term "refined" refers to a food that has been heavily processed. Fiber and bran have been stripped away, along with many of the vitamins and minerals they contain. As a result, the body processes these carbs quickly, and they supply little nutrition while having similar calorie counts. Enrichment refers to the addition of vitamins and minerals to refined foods in order to boost their nutritional value. Obesity and diabetes are more likely in people who eat a diet high in simple or refined carbohydrates.
When humans consume more carbs than they require at the time, the body stores part of them (as glycogen) in their cells and turns the remainder to fat. Glycogen is a complex carbohydrate that the body can convert to energy quickly and easily. Glycogen is a type of carbohydrate that is stored in the liver and muscles. During periods of severe exertion, muscles consume glycogen for energy. The amount of carbohydrates stored as glycogen is nearly enough for a day's worth of calories. Carbohydrates are stored in a few different human tissues as complex carbohydrates that cannot be used for energy.
Carbohydrates should account for roughly 50 to 55 percent of total daily calories, according to most experts. Added sugars should account for no more than 10% of total daily calories. Syrups and other caloric sweeteners used in other food products are referred to as added sugars. Added sugars are included on food labels as an ingredient. Brown sugar, corn syrup, dextrose, fructose, glucose, high-fructose corn syrup, honey, invert sugar, lactose, malt syrup, maltose, molasses, raw sugar, sucrose, trehalose, and turbinado sugar are among them. Sugars found naturally in foods like fruit and milk are not added sugars.
Glycemic index
The glycemic index of a carbohydrate measures how quickly it raises blood sugar levels after eating. The values range from 1 (the slowest) to 100 (the fastest) (the fastest, the index of pure glucose). However, the rate at which the level rises is affected by what other foods are consumed at the same time as well as other factors.
Complex carbs have a lower glycemic index than simple carbs, however there are exceptions. Fructose (the sugar found in fruits), for example, has no effect on blood sugar levels.
The glycemic index of a food is also affected by the following factors:
- Processing: Foods that have been processed, refined, or finely milled have a higher glycemic index.
- Type of starch: Different starches are absorbed in different ways. Potato starch, for example, is quickly digested and absorbed into the bloodstream. Barley is more slower to digest and absorb than wheat.
- Fiber content: The more fibre in a food, the more difficult it is to digest. Sugar is taken more slowly into the bloodstream as a result.
- Ripeness of fruit: The more sugar a fruit has, the higher its glycemic index will be.
- Fat or acid content: The slower a food is digested and the slower its carbohydrates are taken into the bloodstream, the more fat or acid it contains.
- Preparation: The speed with which a food is taken into the bloodstream is influenced by how it is cooked. Cooking or grinding a food raises its glycemic index since these activities make it simpler to digest and absorb the food.
Other factors: The pace at which carbs are converted to sugar and absorbed varies depending on how the body processes food. The speed with which a dish is chewed and swallowed has an impact as well.
The glycemic index is regarded to be significant because carbohydrates that quickly raise blood sugar levels (those with a high glycemic index) also raise insulin levels quickly. Low blood sugar (hypoglycemia) and hunger are common side effects of a rise in insulin, which can lead to overeating and weight gain.
Carbohydrates with a low glycemic index do not raise insulin levels nearly as much as those with a high glycemic index. As a result, people are more satisfied after eating. Consuming carbohydrates with a low glycemic index tend to result in healthier cholesterol levels and lowers the risk of obesity and diabetes mellitus, as well as the risk of diabetes complications in persons who already have diabetes.
Using the index to selecting meals does not automatically lead to a healthy diet, despite the link between low glycemic index meals and better health. The glycemic index of some unhealthy foods, such as potato chips and candy bars, is lower than that of other healthy ones, such as brown rice. Vitamins and minerals are found in some foods with a high glycemic index. As a result, this index should only be used as a broad guide to dietary selections.
Glycemic load
Only how rapidly carbohydrates in a diet are taken into the bloodstream is measured by the glycemic index. It excludes the amount of carbohydrate in a food, which is also significant. The glycemic index and the amount of carbohydrate in a food make up the glycemic load. Carrots, bananas, watermelon, and whole-wheat bread, for example, have a high glycemic index but a low glycemic load because they contain very little carbohydrate. These foods have a negligible influence on blood sugar levels.
Glycemic load also considers how the combination of foods consumed together affects blood sugar levels. The glycemic index, on the other hand, does not.
3.13.2 Fats:
Fatty acids and glycerol combine to form fats, which are complex compounds. Fats are required by the body for growth and energy. They're also used to make hormones and other compounds necessary for the body's functions (such as prostaglandins).
Fats are the slowest source of energy, yet they are also the most energy-efficient. Fat provides the body with around 9 calories per gramme, more than twice as many as proteins or carbohydrates. The body stores any surplus energy as fat because lipids are such an efficient type of energy. When the body requires additional energy, it stores additional fat in the abdomen (omental fat) and under the skin (subcutaneous fat). Excess fat can also be stored in blood arteries and organs, where it can obstruct blood flow and damage organs, resulting in serious health problems.
Fatty acids
The body can manufacture (synthesise) specific fatty acids when they are needed. Others, known as essential fatty acids, are unable to be produced and must be obtained through diet. Essential fatty acids account for around 7% of total fat consumption and 3% of total calories in a typical diet (about 8 grams). Linoleic and linolenic acids, which are found in some vegetable oils, are among them. Linolenic acid can be used to make fatty acids like eicosapentaenoic acid and docosahexaenoic acid, which are important for brain development. They are, however, found in some marine fish oils, which constitute a more efficient source.
Omega-6 fatty acids include linoleic acid and arachidonic acid. Omega-3 fatty acids include linolenic acid, eicosapentaenoic acid, and docosahexaenoic acid. Omega-3 fatty acid-rich foods may lower the risk of atherosclerosis (including coronary artery disease). Omega-3 fatty acids are abundant in lake trout and several deep-sea fish. People in the United States consume too many omega-6 fatty acids, which are found in many processed foods' oils, but not enough omega-3 fatty acids. (Women who are pregnant or breastfeeding should pick mercury-free fish.) For further information, see Mercury in Seafood.)
Kinds of fat
There are several types of fat:
- Monounsaturated
- Polyunsaturated
- Saturated
Saturated fats have a higher proclivity for raising cholesterol levels and increasing the risk of atherosclerosis. Saturated fats, which are solid at room temperature, are typically found in animal-derived foods. Monounsaturated and polyunsaturated fatty acids, which are liquid at room temperature, are often found in plant fats. The exceptions are palm and coconut oil. They have a higher percentage of saturated fats than other plant oils.
Trans fats (also known as trans fatty acids) are a type of fat. They are created by adding hydrogen atoms to monounsaturated or polyunsaturated fatty acids (hydrogenation). Hydrogenated fats can be partially or completely hydrogenated (or saturated with hydrogen atoms). In the United States, partly hydrogenated vegetable oils, which are included in many commercially prepared foods, are the most common source of trans fats. Trans fats have been shown to raise cholesterol levels in the body and increase the risk of atherosclerosis.
Fat in the diet
The majority of authorities advise that
• Fat should account for no more than roughly 28% of total daily calories (or fewer than 90 grammes per day).
• Saturated fats should be kept to fewer than 8% of total calories.
It is suggested that trans fats be eliminated from one's diet. Monounsaturated and polyunsaturated fats, especially omega-3 fats, should be exchanged for saturated and trans fats wherever possible.
High-cholesterol individuals may need to lower their overall fat intake even further.
3.13.3 Tests of Carbohydrates, Fats and Proteins in Given Food Stuffs:
Carbohydrates provides energy to cells in the body. Protein is the major functional and structural component of all the cells of the body. Fats and oils are the highest energy sources contain fatty acids which is essential for health which is not produced by the human body.
Aim:
To determine whether the given food items contain carbohydrates, lipids, or proteins.
Theory:
The presence of carbohydrates, fats and proteins in any food stuff is detected by performing the tests for proteins, fats and carbohydrates with the extract of the foodstuff. The benefit is that these exams do not conflict with one another.
Test for Carbohydrates:
- Molisch’s test – Given sample food + Molisch’s reagent → Purple or violet ring confirms the presence of carbohydrate.
- Fehling’s test – Given sample food + Fehling’s reagent → Red precipitate confirms the presence of carbohydrates
- Benedict’s test – Given sample food + Benedict’s reagent → Red precipitate confirms the presence of carbohydrates.
- Tollen’s test – Given sample food + Tollen’s reagent → Silver mirror confirms the presence of carbohydrates.
- Iodine test – Given sample food + Iodine solution → Blue colour solution confirms the presence of starch.
Test for Proteins:
- Biuret test – Given sample food + Aqueous copper sulfate → Violet colouration confirms the presence of Proteins
- Xanthoproteic test – Given sample food + Nitric acid → Yellow colour solution confirms the presence of proteins.
- Millions test – Given sample food + Mercuric sulfate in the presence of sodium nitrite and sulfuric acid → Brick red colour solution confirms the presence of proteins.
- Ninhydrin test – Given sample food + Pyridine solution of ninhydrin → Violet colour solution confirms the presence of proteins.
Test for Oils and Fats:
- Solubility test – Given sample food + Chloroform or alcohol → Miscible with chloroform and immiscible with water the fat presence is confirmed.
- Translucent spot test – Given sample food + rubbed between the folds of filter paper → presence of translucent spot then the presence of fats is confirmed.
- Acrolein test – Given sample food + Potassium bisulfite KHSO4 → Pungent irritating odor then the presence of fats or oil is confirmed.
Materials Required:
- Molisch’s reagent
- Fehling’s reagent
- Benedict’s reagent
- Tollen’s reagent
- Iodine solution
- Copper sulfate solution
- Sodium hydroxide
- Nitric acid
- Mercuric sulfate
- Sodium nitrite
- Alcohol
- Chloroform
- Filter paper
- Potassium bisulfate
- Concentrated hydrochloric acid
- Furfural solution
- Test tubes
- Test tube holder
- Water bath
- Dropper
- Stirrer
- Bunsen burner
Apparatus Setup:
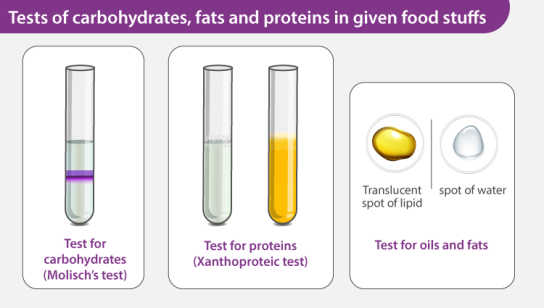
Procedure:
To determine whether a food sample contains carbs, proteins, lipids, or oils, an extract of the food should be made first. After grinding the specified foodstuff, dry it in a mortar with a pestle or by boiling it with a tiny amount of water and extracting it with a tiny amount of an organic solvent.
The following are some examples of how to make a food extract.
- For potatoes – Cut into slices and bring to a boil in water.
- For butter – directly test
- For grapes – Take out the juice.
- For boiled egg – Using water, saturate the white portion of the grind.
Observation and Inference:
Food stuff | For carbohydrates | For proteins | For oils and fats | ||||||||
Butter |
|
|
|
|
|
|
|
|
|
|
|
Potatoes |
|
|
|
|
|
|
|
|
|
|
|
Butter |
|
|
|
|
|
|
|
|
|
|
|
Boiled egg |
|
|
|
|
|
|
|
|
|
|
|
Results and Discussions:
The given food sample contains _________ (carbohydrates/ proteins/ fats).
Precautions:
1. To test the sample meal, always use a little amount of reagents that are freshly prepared.
2. Remove the reagents from the bottle with droppers.
3. During the experiment, wear lab aprons and hand gloves.
3.14.1 Calorific Value Definition:
The amount of heat energy present in food or fuel is calculated by full combustion of a defined quantity at constant pressure and under normal conditions. Calorific power is another name for it. The kilojoule per kilogramme, or KJ/Kg, is the unit of calorific value.
In the combustion process, water vapour is produced, and the heat should be recovered using specified ways. It has a high calorific value if the heat contained in the water vapour can be retrieved. When the heat contained in water vapour cannot be retrieved due to its poor calorific value.
The calorific value of a fuel or meal determines its efficiency. If the value is high, then the efficiency will be high as well. If the value is low, the efficiency will suffer as well. The efficiency of a product is directly related to its calorific value.
3.14.2 Calorific Value of Fuel
The following is a comprehensive list of fuels and their calorific values:
Fuel | Calorific Value Of Fuel (Approximately) |
Cow Dung | 8000 |
Wood | 22000 |
Coal | 33000 |
Biogas | 40000 |
Diesel | 45000 |
Kerosene | 45000 |
Petrol | 45000 |
Methane | 50000 |
LPG | 55000 |
When compared to diesel, petrol, and kerosene, LPG has a higher calorific value.
A detailed list of foods and their calorific values:
The calorific value of food refers to the total amount of energy that a human body can produce during metabolism, which is measured in Kilojoules per 100 grammes or 100 millilitres. Food's calorific value is usually stated in kilocalories (kcal).
The type of food and its calorific value are shown in the table below:
Category of food | Quantity (Grams) | Calorific value (Approximately) | |
Kilojoules (KJ) | Kilocalories (Kcal) | ||
Dietary fibre | 1 | 8 | 2 |
Polyhydric Alcohols | 1 | 10 | 2.4 |
Alcohols | 1 | 29 | 7 |
Carbohydrates | 1 | 17 | 4 |
Protein | 1 | 17 | 4 |
Fats | 1 | 37 | 9 |
When compared to carbs, protein, alcohols, roughage, and other macromolecules involved in the maintenance and metabolic processes, fats have a higher calorific value.
3.14.3 Importance of Calorific Value
It is critical to understand the calorific value of fuel in order to carry out our daily activities. This information aids us in calculating the amount of energy we carry. This information is required by gas shippers and suppliers in order to bill gas customers. It also aids in the calculation of gas shippers' and suppliers' transportation costs.
To carry out regular activities, the human body need calories. The body would stop operating and perish if it didn't have enough calories. People could live a healthy lifestyle if they simply consumed a certain number of calories each day. Too much or too little calorie consumption might lead to health issues.
3.14.4 Caloric Value of Food
The amount of energy stored in dietary substances (proteins, fats, and carbohydrates); the energy value of foodstuffs, measured in calories (cal) or kilocalories (kcal). The notion is applied in a variety of contexts, including food product comparisons and diet planning.
The existence of unoxidized carbon and hydrogen atoms determines the caloric value. A molecule of fat has more unoxidized carbon and hydrogen atoms than a carbohydrate or protein molecule. One gramme of fat has 9.3 kcal (1 kcal = 4.1868 103 joules), while one gramme of carbohydrate contains 4.1 kcal and one gramme of protein has 4.1 kcal. The caloric value (in kcal) of several items (per 100 g) is as follows: scallions, 21; fresh cucumbers, 15; potatoes, 89; cabbage, 27; carrots, 36; apples, 48; lemons, 41; cepes, 32; walnuts, 612; rye bread, 204; and sugar, 390 Food caloric values must be understood in order to calculate rations, which are based on the energy expenditures of people of various jobs, sexes, and ages.
The caloric value is used in animal husbandry to compare the nutritional content of feeds, to create feeding standards for animals, and to plan feed requirements.
Most meals have a calorie value of 4.0-4.5 Meal per 1 kilogramme of dry content. The digestibility of a feed and the absorbability of the digestible elements determine the feed's practical caloric value for the animal.
3.15.1 Carbohydrate and Calorie Content of Foods by Item:
Carbohydrates should be the primary dietary sources for you or your kid athlete, with whole-grain products, veggies, and fruits accounting for three-quarters of the FDA's MyPlate food guide system.
Studies have shown that adequate dietary carbohydrates must be consumed on a daily basis, especially after exercise, to restore levels of carbohydrates (glycogen) stored in the body's muscles and liver, which, as the preferred fuel for most types of exercise, is required for peak athletic performance. The lesson from this research is clear: not only do you need a diet rich in nutrient-dense carbohydrates, but you also need extra carbohydrate. Glycogen depletion causes weariness and poor performance, which can be avoided by eating a carbohydrate-rich diet and taking periodic rest days to allow the muscles to recover glycogen.
3.15.2 Calories and health
To survive, the human body need calories. The body's cells would perish without energy, the heart and lungs would cease beating, and the organs would be unable to carry out the basic functions required for survival. Food and drink provide this energy to people.
People would most likely live healthy lives if they merely consumed the number of calories required each day. Calorie intake that is either too low or too much will eventually cause health issues.
The amount of potential energy in food is determined by the number of calories it contains. The substance from which the calories are derived is just as significant as the calories themselves.
The calorific values of three major dietary components are listed below:
- 1 g of carbohydrates contains 4 kcal
- 1 g of protein contains 4 kcal
- 1 g of fat contains 9 kcal
As an example, here is the breakdown of how a person would get calories from one cup of large eggs Trusted Source, weighing 243 g:
Fat: 23.11 g
23.11 g x 9 kcal = 207.99 kcal
Protein: 30.52 g
30.52 x 4 kcal = 122.08 kcal
Carbohydrate: 1.75 g
1.75 x 4 kcal = 7 kcal
243 g of raw egg contains 347 kcal. 208 kcal comes from fat, 122 kcal is taken from protein, and carbohydrate provides 7 kcal.
3.15.3 HOW THE CALORIE CONTENT OF FOOD IS DETERMINED:
Let's start by making sure everyone knows what a calorie is. A calorie is just the amount of energy required to elevate 1 gramme of water by one degree Celsius at standard atmospheric pressure. When it comes to eating calories, this makes sense. Food gives us energy, which our bodies require to perform throughout the day. While composing this response, I'm trying to burn roughly 100 calories. If not, this 100-calorie snack pack will not be as healthy as I had hoped and will end up on my hips!
Second, it's vital to remember that food calories are calculated in kilocalories, which means that for every 1 calorie listed, 1000 actual calories are consumed.
A "bomb calorimeter" was once used by manufacturers to calculate calories. The food supply was placed in a sealed container filled with water during this operation. They would then use electrical energy to burn the food. They would test the temperature of the water after the food had completely burnt off to see how many degrees it had been elevated and hence how many calories had been consumed.
The FDA began forcing food producers to label the amounts of nutrients and calories contained in their goods in 1990, under the Nutrition Labeling and Education Act of 1990. As a result of this act, the bomb calorimeter method for calculating the number of calories in food was abandoned. One rationale for this was to make meeting the standard easier and less expensive for manufacturers. Another advantage of the bomb calorimeter approach is that it measures all of the product's calories. Because most diets contain indigestible components, like as fibre, that pass through our system and are expelled in the form of bum brownies, the bomb calorimeter would consistently overestimate consumed calories. Instead, they adopted the Atwater technique, which is a simpler way.
Nutrition Facts
Serving Size 5 pieces (41g) / Servings Per container 3
Amount per Serving |
|
Calories 210 | Calories from Fat 100 |
| %Daily Value* |
Tital Fat 11g | 17% |
Saturated Fat 5g |
|
Trans fat | 0g |
Cholesterol <5mg | 0% |
Sodium | 50 mg |
Total Carbohydrates | 26g |
Dietary fiber 1g | 4% |
Sugar 18g |
|
Proteins 3g |
|
Vitamin A | 0% | Vitamin C | 0% |
Calcium | 8% | Iron | 10% |
*Percent Daily values are based on 2000 calories diet. Your daily values may be higher or lower depending on your calorie needs:
| |||
| Calories | 2000 | 2500 |
Total Fat | Less than | 65g | 80g |
Sat Fat | Less than | 20g | 25g |
Cholesterol | Less than | 300 mg | 300mg |
Sodium | Less than | 2,400 mg | 2,400 mg |
Total Carbohydrates |
| 300mg | 375 mg |
Dietary Fiber |
| 25g | 30g |
3.15.4 Sample Problem: Calculating Specific Heat
Use the information in this nutrition facts label to determine the amount of Calories (Cal = kcal) and kilojoules (kJ) from fat, carbohydrates and protein in the snack mix.
Step 1: List the known quantities and plan the problem
Known
Energy per gram
- Fat = 9 kcal/g (Cal/g)
- Carbohydrate = 4 kcal/g (Cal/g)
- Protein = 4 kcal/g (Cal/g)
Grams
- Fat = 11 g
- Carbohydrate = 12 g
- Protein = 5 g
The label specifies the number of grammes of each energy source (fat, carbohydrate, and protein) as well as the amount of energy per gramme of each energy source. To get the Energy, multiply the grammes by the Energy per gramme. The conversion factor is the energy per gramme. Because you want your final answer to be energy in Cal, kcal, or kJ, it should be multiplied such that the energy is on top and the grammes are on the bottom.
Step 2: Solve
Energy from Fat:
11 g x 9 kcal/g = 99 kcal = 99 Cal
Convert to kJ
99 Cal x 4.184kJ/Cal = 414.216 kJ= 414kJ
Energy from Carbohydrate:
12 g x 4 kcal/g = 48 kcal = 48 Cal
Convert to kJ
48 Cal x 4.184kJ/Cal = 200.832 kJ = 201 kJ
Energy from Protein:
5 g x 4 kcal/g = 20 kcal = 20 Cal
Convert to kJ
20 Cal x 4.184kJ/Cal = 83.68 kJ = 84 kJ
Step 3: Think about your result.
When these three sources of energy are evaluated for the snack item in this example, the majority of the energy comes from fat first, then carbohydrates, and finally protein.