Module 02
Spectroscopic techniques and applications
Q-1 What are IR spectroscopy?
Answer:
The change in the vibrational motion of molecule is absorbed in the Infrared Region this is why it is also called as the Infrared Spectroscopy. If vibration in the molecule persists then it should change its dipole moment that may be the magnitude form or oriented with its direction. When taking the example of Polar molecule (A-B) as the polar molecule shows the property of the high electronegative atom attracts the electron pair towards it so that it gets partial negative and partial positive charges. So in the polar molecule the atoms are making rotation therefore one side is positive pole while other is negative but due to the rotation at its own axis then there is no change in the magnitude effects hence it posses the generation of magnetic field which in result there in the electric flux or there is a change in the direction.
Q-2Explain the applications of spectroscopy.
Answer:
Applications of Spectroscopy:
- Cure monitoring of composites using optical fibers.
- Estimate weathered wood exposure times using near infrared spectroscopy.
- Measurement of toxic compounds in blood samples
- Non-destructive elemental analysis by X-ray fluorescence.
Q-3 Explain selection rule of spectroscopy and its applications.
Answer:
On the irradiation of the sample with infrared electromagnetic radiation then its frequency can be measured by the Infrared spectroscopy. It is a technique used to study the vibrations between atoms because atomic vibrational excitations occur in the infrared region of the electromagnetic spectrum. The bonds between atoms can be thought of as a spring connecting two masses. In the spring-mass analogy the moving system can be approximated by a simple harmonic oscillator. The frequency oscillation is proportional to
√k/m
Where,
k is the spring constant
m is the mass of the object.
Applications:
1-Infrared technique is used in organic and inorganic chemistry.
2-This is used in quality control, dynamic measurement.
3-This is majorly used in the forensic labs.
4-Infrared Spectroscopy is used in measuring the degree of polymerization in manufacturing the polymers.
Q-4Explain the structure of fluorescence and its properties.
Answer:
Fullerene is a soccer ball like structure which is hollow from inside and is polymorphic in nature (arrange in hexagon and pentagon form) These are arranged in manner that posses 2 hexagon share a common wall while 2 different pentagon never share the common wall. Each carbon atom is sp2 hybridized. Electric spark is produced at graphite electrode at inert atmosphere and low pressure which gives back black soot that consist of fullerene and impurities. These impurities are removed by the method of sublimation.
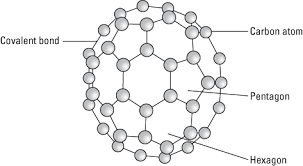
Properties:
All carbon atoms are sp2 hybridized with FCC in nature. This is mustard yellow in color. The fullerene may be Exohederal or Endohederal structure based on the occupying the space.
Q-5Explain NMR in brief.
Answer:
NMR stands for the Nuclear Magnetic Resonance Spectroscopy. NMR Spectroscopy technique is used to observe local magnetic fields around atomic nuclei. An NMR instrument allows the molecular structure of a material to be analyzed by observing and measuring the interaction of nuclear spins when placed in a powerful magnetic field. For the analysis of molecular structure at the atomic level, electron microscopes and X-ray diffraction instruments can also be used, but the advantages of NMR are that sample measurements are non-destructive and there is less sample preparation required.
Nuclear magnetic resonance (NMR) spectroscopy is a crucial analytical tool for organic chemists. The research in the organic lab has been significantly improved with the aid of the NMR. Not only can it provide information on the structure of the molecule, it can also determine the content and purity of the sample. Proton (1H) NMR is one of the most widely used NMR methods by organic chemists. The protons present in the molecule will behave differently depending on the surrounding chemical environment, making it possible to elucidate their structure.
A spinning charge generates a magnetic field that results in a magnetic moment proportional to the spin. In the presence of an external magnetic field, two spin states exist; one spin up and one spin down, where one aligns with the magnetic field and the other oppose it.
Chemical shift is characterized as the difference between the resonant frequency of the spinning protons and the signal of the reference molecule. Nuclear magnetic resonance chemical change is one of the most important properties usable for molecular structure determination. There are also different nuclei that can be detected by NMR spectroscopy, 1H (proton), 13C (carbon 13), 15N (nitrogen 15), 19F (fluorine 19), among many more. 1H and 13C are the most widely used. The definition of 1H as it is very descriptive of the spectroscopy of the NMR. Both the nuts have a good charge and are constantly revolving like a cloud. Through mechanics, we learn that a charge in motion produces a magnetic field. In NMR, when we reach the radio frequency (Rf) radiation nucleus, it causes the nucleus and its magnetic field to turn (or it causes the nuclear magnet to pulse, thus the term NMR).
Q-6Explain MRI and its mechanism.
Answer:
This is the medical imaging technique which is used in radiology to form pictures of anatomy and physiological process of body. This technique uses very strong magnetic fields and high radio waves to generate organs image. MRI is a medical application of NMR that can also be used for imaging in other NMR applications such as NMR spectroscopy.
Mechanism
Magnetic resonance imaging (MRI) uses the body's natural magnetic properties to produce detailed images from any part of the body. For imaging purposes the hydrogen nucleus (a single proton) is used because of its abundance in water and fat. The hydrogen proton can be likened to the planet earth, spinning on its axis, with a north-south pole. In this respect it behaves like a small bar magnet. Under normal circumstances, these hydrogen proton “bar magnets” spin in the body with their axes randomly aligned.
When the body is placed in a strong magnetic field, such as an MRI scanner, the protons' axes all line up. This uniform alignment creates a magnetic vector oriented along the axis of the MRI scanner. MRI scanners come in different field strengths, usually between 0.5 and 1.5 tesla.
When additional energy is added to the magnetic field, the magnetic vector is deflected. The radio wave frequency (RF) that causes the hydrogen nuclei to resonate is dependent on the element sought and the strength of the magnetic field.
The strength of the magnetic field can be altered electronically from head to toe using a series of gradient electric coils, and, by altering the local magnetic field by these small increments, different slices of the body will resonate as different frequencies are applied. When the radiofrequency source is switched off the magnetic vector returns to its resting state, and this causes a signal (also a radio wave) to be emitted. It is this signal which is used to create the MR images. Receiver coils are used around the body part in question to act as aerials to improve the detection of the emitted signal. The intensity of the received signal is then plotted on a grey scale and cross sectional images are built up.Multiple transmitted radiofrequency pulses can be used in sequence to emphasise particular tissues or abnormalities. A different emphasis occurs because different tissues relax at different rates when the transmitted radiofrequency pulse is switched off. The time taken for the protons to fully relax is measured in two ways. The first is the time taken for the magnetic vector to return to its resting state and the second is the time needed for the axial spin to return to its resting state. The first is called T1 relaxation, the second is called T2 relaxation.
An MRI examination is thus made up of a series of pulse sequences. Different tissues have different relaxation times and can be identified separately. By using a “fat suppression” pulse sequence, for example, the signal from fat will be removed, leaving only the signal from any abnormalities lying within it.
Most diseases manifest themselves by an increase in water content, so MRI is a sensitive test for the detection of disease. The exact nature of the pathology can be more difficult to ascertain: for example, infection can in some cases look similar. A careful analysis of the images by a radiologist will often yield the correct answer.
There are no known biological hazards of MRI because, unlike x ray and computed tomography, MRI uses radiation in the radiofrequency range which is found all around us and does not damage tissue as it passes.
Q-7what is diffraction?
Answer:
Diffraction:
The aim of this chapter is the presentation of neutron diffraction as a tool to elucidate the structure of condensed matter, in particular crystalline matter. It is meant as an general introduction for the widespread scientific public from all fields of geosciences, chemistry, materials sciences, metallurgy and mechanical engineering, potentially involved in the characterization and elaboration of materials for energy production, conversion, transport and storage, potentially interested in application of neutron diffraction to their problems. It is not intended to satisfy all – i.e., mathematical – exigencies of a full blown condensed matter physicist or a pure crystallographer. Throughout this chapter one is faced with the eternal problem whether one should start with the egg or with the hen. One could start with some crystallographic notions and introduce then the diffraction tool; one could start with wave functions and derive cross-sections later. There is not a single way, not a single order to understand everything, the diffraction tool in its different declinations and the objects probed by this technique. Therefore I started with an easy mechanistically approach to scattering and diffraction, before diving into a more involved treatment necessary to prepare for the full scope of the method. Then, some crystallographic notions are introduced, which are absolutely necessary to deal with diffraction experiments, before one dives once again in a more complex treatment leading to some specific “neutron issues”. Finally, a toolbox is presented making the interpretation of down-to-earth diffraction experiments approachable for scientist in need of this powerful technique. In this approach it was unavoidable to use some terms, e.g., that of “unit cell”, to some extend in some context before introducing them properly in another context. An index at the end of this chapter may help the reader to fill gaps if needed. As diffraction is commonly considered as a special case of scattering, one should start with a general introduction and definition of scattering.
Q-8what are scattering?
Answer:
Scattering is the deflection of radiation, rather, propagating waves) from a straight trajectory by one (bending) or more paths (spreading) when encountering localized non-uniformities, i.e., an object, in the medium through which it passes. All kinds of waves may be scattered, e.g., sound waves, light waves, or particle waves. We may well consider the deflection as caused by collisions if we consider the moving particle character of the propagating wave, particularly appropriate in the case of neutrons, and if we consider other particles, such as the atoms of crystalline matter, as the “localized non-uniformities”. The examples are manifold: cosmic rays are scattered by the Earth’s atmosphere, electrons by gas atoms in fluorescent lamps and neutrons by the moderator in a nuclear reactor. Scatterers can be elementary particles, all kinds and scales of density fluctuations in condensed matter (bubbles in liquids, droplets in aerosols, pores in solids), defects in crystals and atoms in condensed matter, roughness of surfaces, fibers, etc. The term “scattering” includes as well a deviation of reflected radiation from the angle predicted by the laws of reflection and refraction. Reflections undergoing scattering are called diffuse reflections, whereas un-scattered reflections are called specular (mirror like) reflections. The degree of diffuse reflection of light depends on the surface roughness; it is caused by surface irregularities smaller than the wavelength of the incident light.
Q-9What are emission spectroscopy?
Answer:
Atoms or molecules that are excited to high energy levels can decay to lower levels by emitting radiation. For atoms excited by a high-temperature energy source this light emission is commonly called atomic or optical emission and for atoms excited with light it is called atomic fluorescence. The decay of the high energy levels to the lower levels by radiation emission is called as emission spectroscopy.
Q-10Explain Raman spectroscopy.
Answer:
Raman Spectroscopy deals with the analysis of the chemical in a non-destructive form. This help in extracting a detailed information about the chemical structure, phase and polymorphy, crystallinity and molecular interactions. This is purely based on the interaction of light with chemical bonds in the material.
Raman is used to be work as a scattering technique where by the it help in scattering the light of the source at different wavelength while some of the scattered lights are of same wavelength as of source and hence that light are not useful for gathering the further information this is called as the Rayleigh Scatter while the remaining light that is scattered at different wavelength are called as the Raman Scatter.