Unit - 1
Introduction
Q1) What is geology explain in brief.
A1)
- Geology provides a systematic knowledge of construction materials and their occurrence, formation, durability, strength, hardness, and uses. Before starting any major/minor civil construction at a place, a detailed geological report which is accompanied by geological maps and sections is prepared.
- The detailed geological report contains types of rocks (Petrology), types of formations (geological structures), and physical properties of the earth(Geophysics). Petrology is the study of rocks, where it provides rock hardness, chemical composition, strength, durability, etc.
- Petrology is particularly important as it gives the required load-bearing properties of the rock which will help in deciding the usage. Sometimes there is a possibility of rocks of acceptable compressive strength being susceptible to chemical reactions, and may not be preferred for construction in certain fields.
- Structural Geology is the study of patterns that are formed below the earth like folds, faults, joints, and unconformity. Structural Geology is the necessary factor at present for major construction projects. On account of the effects of these anomalies on the structures, there are few examples with negligible geological considerations which create loss to both life and property.
- In recent years it has been noticed that more importance is given to the study of geological structures due to past experiences. Geophysics is the study of the physical properties and composition of the interior earth using a gravity field, magnetic field, and geothermal field. Modern geophysics methods that are used in civil engineering are mainly non-destructive testing.
- Equipment like geophones is employed to map the interiors of the earth's crust by creating vibrations at a certain point and recording the same at a particular distance. Geophones use the concept of wave propagation to map the materials that may be present in the field. Geophysics is particularly important for shallow constructions where the underground amenities are not known.
Q2) Explain importance of geology in civil engineering.
A2)
The importance of geology in civil engineering may be briefly outlined as follows:
- Geology provides a systematic study of the structure and properties of construction materials and their occurrence. Civil engineers need to know the properties of rocks accurately to enable them to consider different rocks for any required purpose i.e. as a foundation rock, as road metal, as concrete aggregate, as building stones, as the roofing material for decorative purposes.
- The selection of a site is important from the viewpoint of stability of foundation and availability of construction materials. Geology provides knowledge about the site used in the construction of buildings, dams, tunnels, tanks, reservoirs, highways, and bridges.
- Geology helps to identify areas susceptible to failures due to geological hazards such as earthquakes, landslides, weathering effects, etc.
- Knowledge about the nature of the rocks is very necessary for tunneling, constructing roads, and determining the stability of cuts and slopes.
- The foundation problems of dams, bridges, and buildings are directly related to the geology of the area where they are to be built.
- The knowledge of groundwater is necessary for connection with excavation works, water supply, irrigation, and many other purposes. Hydrological maps provide information about the distribution of surface water channels and the groundwater depth.
- Geological maps help in planning civil engineering projects. It provides information about the structural deposition of rock types in the proposed area.
- Geology helps in determining the earthquake-prone areas. If any geological features like faults, folds, etc. are found, they have to be suitably treated to increase the stability of the structure.
- The knowledge of erosion, transportation, and deposition (ETD) by surface water helps soil conservation, river control, coastal and harbor works.
- A geological survey of a site before starting a project will reduce the overall cost.
Q3) Explain scope of geology in civil engineering and geology in construction field.
A3) Scope of geology in civil engineering:
- It is defined as that of applied science which deals with the application of geology for a safe, stable, and economic design and construction of a civil engineering project.
- Engineering geology is almost universally considered as essential as that of soil mechanics, the strength of the material, or the theory of structures.
- The application of geological knowledge in planning, designing, and construction of big civil engineering projects.
- The basic objects of a course in engineering geology are two folds.
- It enables a civil engineer to understand the engineering implications of certain condition should relate to the area of construction which is essentially geological.
- It enables a geologist to understand the nature of the geological information that is essential for a safe design and construction of civil engineering projects.
- The scope of geology can be studied regarding major activities of the profession of a civil engineer which are Construction, Water resources development, and Town and regional planning
Geology in the construction field
- Topographic Maps: It gives details of relief features and understands the relative merits and demerits of all the possible sides of a proposed structure.
- Hydrological maps: This map gives broad details about the distribution and geometry of the surface of the water channel.
- Geological maps: The petrological characters and structural disposition of rock types gives an idea about the availability of construction materials.
Q4) Give the brief discussion on geological survey of India.
A4) Geological Survey of India (GSI)
- Geological Survey of India (GSI) Established in 1851, started its voyage to investigate for and assess coal and other mineral resources of the country with regional level exploration. In the 160 years since its foundation, GSI has continued to grow and diversify into various geoscientific activities and delivered impeccable contributions in the arena of geosciences.
- After independence, GSI‟s activities in mineral exploration, as well as baseline surveys, have increased manifold to sustain the momentum of national economic development and to meet the increasing demands of various stakeholders.
- Over the years, it has not only developed into a huge repository of precious geoscientific data applied in various developmental sectors in the country but has also attained the status of a geo-scientific organization of international repute.
- The principal function of GSI relates to the creation and updation of national geoscientific data and mineral resource assessment, air-borne and marine surveys, and conducting multifarious geotechnical, geo-environmental, and natural hazards studies, glaciology, seismotectonic, etc. and to nurture studies on fundamental research.
- In all the developmental facets of the country including coal, steel, cement, metals/ minerals, and power industries, GSI made a neat contribution and remained relevant in the national context.
- The outcome of the work of GSI has immense societal value as well as relevance to global perspective adopting state-of-the-art technologies and using cutting-edge methodologies.
- Functioning and annual programs of GSI assume significance in the national perspective since it is directly related to delivering the public good. With its headquarters in Kolkata, GSI has six Regional offices at Lucknow, Jaipur, Nagpur, Hyderabad, Shillong, and Kolkata and offices in almost all states of the country.
- The Geological Survey of India is an attached office to the Ministry of Mines. The Union Cabinet constituted a High Powered Committee (HPC) to thoroughly review the functioning of the Geological Survey of India and assess its capacity to meet the emerging challenges taking into account the technological and manpower resources of the organization.
- The report of the Committee was submitted in March 2009 and approved by the Union Cabinet in October 2011. The revised organizational structure as proposed by HPC has largely been implemented.
- Activity Domain of GSI The GSI is the prime provider of basic earth science data to the government, industry, and the public, as well as a responsive participant in international geoscientific fora.
- The vibrant steel, coal, metals, cement, and power industries, which expanded phenomenally in the post independence era, bear eloquent testimony to the GSI‟s relevance in the national context. The geoscientific work of GSI encompasses practically the entire gamut of earth sciences and thus great responsibilities are bestowed on the organization.
- Earth science by its very nature is highly multidisciplinary and has immense societal values. To remain relevant for the cause of the society, mankind, global perspective, and its environment, GSI faced challenges of the time to reorient its organizational structure and strengthen its capacity building.
Q5) Give the hpc recommendation on engineering geology.
A5)
Following the HPC recommendations, GSI is executing its programs through Mission-Region hybrid matrix mode with its five Mission offices and three Support Systems.

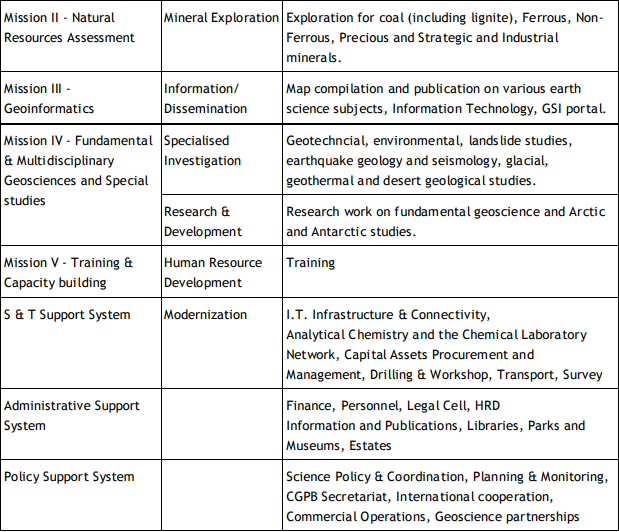
Q6) Explain scope of geological survey of India.
A6) Scope of Geological Survey of India
- Enable and facilitate the providing of objective, impartial, and up-to-date geological expertise and geoscientific information of all kinds, particularly for decision-making for policy, commercial, economic, and societal needs.
- Systematically document the geology and geological processes of the surface and subsurface of India and its offshore areas using the latest and most cost-effective techniques and methodologies, including geophysical and geochemical, and geological surveys.
- Develop and continually enhance GSI’s core competence in survey and mapping through continued accretion, management, coordination, and utilization of spatial databases (including those acquired through remote sensing) and function as a ‘Repository’ or ‘clearing house’ for the purpose and use new and emerging computer-based technologies for dissemination of geoscientific information and spatial data, through cooperation and collaboration with other stakeholders in the Geoinformatics sector.
- Explore (through the ground, airborne, satellite, and marine surveys) and scientifically assess mineral, energy, and water resources for the country and facilitate their optimal exploration through proactive information dissemination.
- Maintain a leadership role in the geological field and develop partnerships with Central, State, and other institutions, to help create enhanced execution capability and capacity in the field of geology in furtherance of GSI’s Vision and the objectives of this Charter.
- Coordinate geoscientific activities with stakeholders in all sectors related to geoscience to help sustainably manage our natural resources, including water.
- Conduct multidisciplinary as well as fundamental Geoscientific research and studies (including geotechnical investigations, physical, chemical, and biological hazard geo-investigations, climate change studies, paleogeo studies, etc.), and foster partnerships with State and Central research and academic institutions for the purpose.
- Actively participate in international collaborative projects to improve our understanding of the earth and its ecosystems and its geology, including studies related to tectonics, global warming and climate change, and Polar studies.
- Generally advance the cause of the geoscience by documentation, propagation, archiving, and education, including creation and management of museums, monuments and parks, archives, libraries, and other facilities for use of students, researchers, and the public. In particular, constantly endeavor to popularize Geoscience at school and university levels through production and dissemination high quality audio-visual and printed material, and through the medium of the Internet. Also, hold exhibitions and special events to bring geoscientific concepts before the public.
Q7) What is national institute of rock mechanics.
A7) National Institute of Rock Mechanics (NIRM)
- The National Institute of Rock Mechanics (NIRM) is a premiere center for research in applied and basic rock mechanics. It is an ISO 9001: 2000 certified research Institute.
- The Institute provides research and consultancy services for improving safety and productivity in the mining and civil engineering sectors. With its rich experience, underpinned by the strength of world-class software and laboratory facilities, NIRM plays a vital role in offering technical services in mining, hydroelectric, and tunneling projects, site evaluation for construction of nuclear power plants, and other infrastructure development projects both in India and abroad. Due to its assured quality of work, the Institute has been receiving several challenging projects from governmental and non-governmental organizations.
- NIRM has been carrying out research work through both government-funded and industry-sponsored S&T and consultancy projects. The Institute has been extending its support to the industry in the following areas :
- Metalliferous mines / Hard Rock Mines
- Coal Mines
- Hydroelectric & Tunnelling Projects
- Other Civil Construction Projects
- The major projects undertaken by NIRM, to mention a few, are the underground cavern for storage of crude oil at Vishakhapatnam, controlled blasting for Bangalore metro project, site evaluation for a nuclear power plant at Kudankulam, construction-stage technical services for a nuclear plant at Rawatbhatta, Rajasthan, DPR stage investigation at Bunakha project, Bhutan, achieving yet another milestone in the annals of NIRM during the year 2010-11.
- During the year 2010-11, 37 projects were completed and 33 were in progress. The finance of the Institute remained satisfactory during the year, by realizing a total cash flow of 8.6 crores. A highly skilled and creative research team of NIRM has contributed 51 technical papers at various national/international journals and proceedings of seminars.
Q8) Explain what is minerals? And what is composition?
A8) Minerals
- Mineral, naturally occurring homogeneous solid with definite chemical composition and a highly ordered atomic arrangement; it is usually formed by inorganic processes. There are several thousand known mineral species, about 100 of which constitute the major mineral components of rocks; these are the so-called rock-forming minerals.
- A mineral, which by definition must be formed through natural processes, is distinct from the synthetic equivalents produced in the laboratory. Artificial versions of minerals, including emeralds, sapphires, diamonds, and other valuable gemstones, are regularly produced in industrial and research facilities and are often nearly identical to their natural counterparts.
- By its definition as a homogeneous solid, a mineral is composed of a single solid substance of uniform composition that cannot be physically separated into simpler chemical compounds. Homogeneity is determined relative to the scale on which it is defined.
- A specimen that appears homogeneous to the unaided eye, for example, may reveal several mineral components under a microscope or upon exposure to X-ray diffraction techniques. Most rocks are composed of several different minerals; e.g., granite consists of feldspar, quartz, mica, and amphibole.
- Also, gases and liquids are excluded by a strict interpretation of the above definition of a mineral. Ice, the solid-state of water (H2O), is considered a mineral, but liquid water is not; liquid mercury, though sometimes found in mercury ore deposits, is not classified as a mineral either.
- Such substances that resemble minerals in chemistry and occurrence are dubbed mineraloids and are included in the general domain of mineralogy.
Composition
- Since a mineral has a definite composition, it can be expressed by a specific chemical formula. Quartz (silicon dioxide), for instance, is rendered as SiO2, because the elements silicon (Si) and oxygen (O) are its only constituents and they invariably appear in a 1:2 ratio. The chemical makeup of most minerals is not as well defined as that of quartz, which is a pure substance. Siderite, for example, does not always occur as pure iron carbonate (FeCO3); magnesium (Mg), manganese (Mn), and, to a limited extent, calcium (Ca) may sometimes substitute for the iron. Since the amount of the replacement may vary, the composition of siderite is not fixed and ranges between certain limits, although the ratio of the metal cation to the anionic group remains fixed at 1:1. Its chemical makeup may be expressed by the general formula (Fe, Mn, Mg, Ca) CO3, which reflects the variability of the metal content.
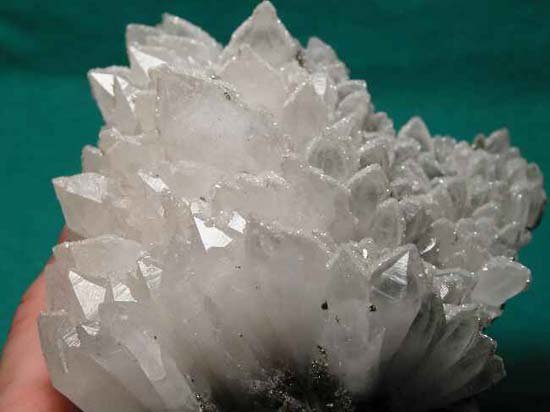
Fig. Trigonal (rhombohedral) crystals of quartz.
- Minerals display a highly ordered internal atomic structure that has a regular geometric form. Because of this feature, minerals are classified as crystalline solids.
- Under favorable conditions, crystalline materials may express their ordered internal framework by a well-developed external form, often referred to as crystal form or morphology. Solids that exhibit no such ordered internal arrangement are termed amorphous. Many amorphous natural solids, such as glass, are categorized as mineraloids.
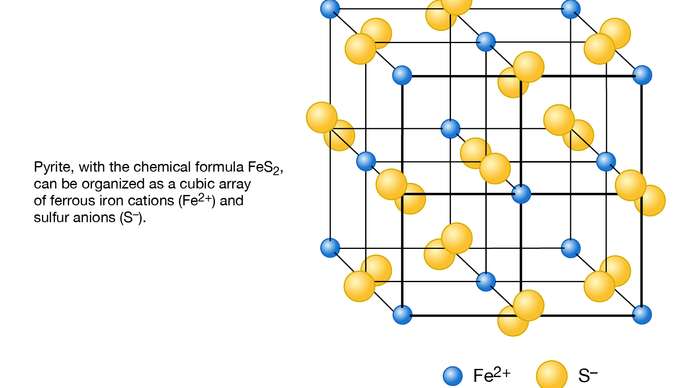
Fig. Schematic representation of the structure of pyrite (FeS2) as based on a cubic array of ferrous iron cations (Fe2+) and sulfur anions (S−).
- Traditionally, minerals have been described as resulting exclusively from inorganic processes; however, current mineralogic practice often includes as minerals those compounds that are organically produced but satisfy all other mineral requirements. Aragonite (CaCO3) is an example of an inorganically formed mineral that also has an organically produced, yet otherwise identical, counterpart; the shell (and the pearl, if it is present) of an oyster is composed to a large extent of organically formed aragonite. Minerals also are produced by the human body: hydroxylapatite [Ca5(PO4)3(OH)] is the chief component of bones and teeth, and calculi are concretions of mineral substances found in the urinary system.
Q9) Give physical properties of minerals.
A9) Physical properties of minerals
- Mineralogists are scientists who study minerals. One of the things mineralogists must do is identify and categorize minerals. While a mineralogist might use a high-powered microscope to identify some minerals, most are recognizable using physical properties.
Color, Streak, and Luster
- Diamonds are popular gemstones because the way they reflect light makes them very sparkly. Turquoise is prized for its striking greenish-blue color. Notice that specific terms are being used to describe the appearance of minerals.
Color
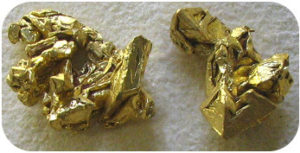
This mineral is shiny, very soft, heavy, and gold in color, and is gold.
- Color is often useful, but should not be relied upon. Different minerals may be the same color. Real gold, as seen in figure 1.3, is very similar in color to the pyrite.
- Additionally, some minerals come in many different colors. Quartz, for example, maybe clear, white, gray, brown, yellow, pink, red, or orange. So color can help but do not rely on color as the determining property. Fig. 1.4 shows one sample of quartz that is colorless and another purple quartz. A tiny amount of iron makes the quartz purple. Many minerals are colored by chemical impurities.
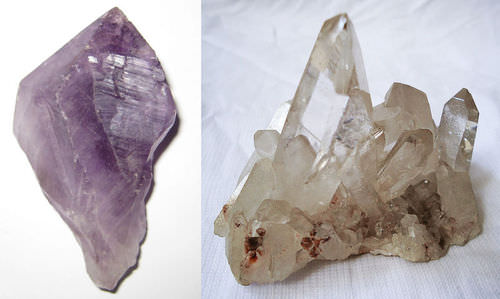
Purple quartz, known as amethyst, and clear quartz are the same mineral despite the different colors.
Luster
- Luster is how the surface of a mineral reflects light. It is not the same thing as color, so it crucial to distinguish luster from color. For example, a mineral described as “shiny yellow” is being described in terms of luster (“shiny”) and color (“yellow”), which are two different physical properties. Standard names for luster include metallic, glassy, pearly, silky, greasy, and dull.
- It is often useful to first determine if a mineral has a metallic luster. A metallic luster means shiny like polished metal. For example cleaned polished pieces of chrome, steel, titanium, copper, and brass all exhibit metallic luster as do many other minerals. Of the nonmetallic clusters, glassy is the most common and means the surface of the mineral reflects light like glass. Pearly luster is important in identifying the feldspars, which are the most common type of mineral.
- Pearly luster refers to a subtle iridescence or color play in the reflected light, the same way pearls reflect light. Silky means reflecting light with a silk-like sheen. Greasy luster looks similar to the luster of solidified bacon grease. Minerals with dull luster reflect very little light. Identifying luster takes a little practice. Remember to distinguish luster from color.
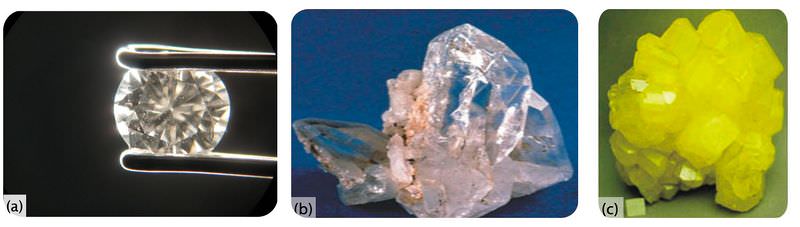
(a) Diamond has an adamantine luster. (b) Quartz is not sparkly and has a vitreous, or glassy, luster. (c) Sulfur reflects less light than quartz, so it has a resinous luster.
Streak
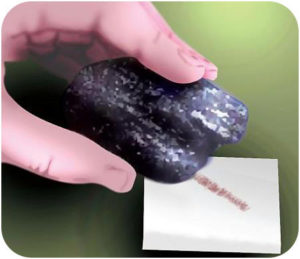
The streak of hematite across an unglazed porcelain plate is red-brown.
Q10) Explain what is specific gravity, cleavage and fracture.
A10) Specific Gravity
- Mass is a measure of the amount of matter in an object. The amount of space an object takes up is described by its volume. The density of an object depends on its mass and its volume. For example, the water in a drinking glass has the same density as the water in the same volume of a swimming pool.
- The specific gravity of a substance compares its density to that of water. More dense substances have higher specific gravity.
- Hardness is the strength with which a mineral resists its surface being scraped or punctured. In working with hand samples without specialized tools, mineral hardness is specified by the Mohs hardness scale.
- The Mohs hardness scale is based on 10 reference minerals, from talc the softest (Mohs hardness of 1), to diamond the hardest (Mohs hardness of 10). It is a relative, or nonlinear, scale. A hardness of 2.5 simply means that the mineral is harder than gypsum (Mohs hardness of 2) and softer than calcite (Mohs hardness of 3).
- To compare the hardness of two minerals see which mineral scratches the surface of the other.
- With a Mohrs scale, anyone can test an unknown mineral for its hardness. Imagine you have an unknown mineral. You find that it can scratch fluorite or even feldspar, but apatite scratches it. You know then that the mineral’s hardness is between 5 and 6. Note that no other mineral can scratch a diamond.
Cleavage and Fracture
- Breaking a mineral breaks its chemical bonds. Since some bonds are weaker than other bonds, each type of mineral is likely to break where the bonds between the atoms are weaker. For that reason, minerals break apart in characteristic ways.
Cleavage
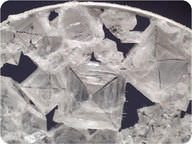
Fig. A close-up view of sodium chloride in a water bubble aboard the International Space Station.
- A mineral that naturally breaks into perfectly flat surfaces is exhibiting cleavage. Not all minerals have cleavage. A cleavage represents a direction of weakness in the crystal lattice.
- Cleavage surfaces can be distinguished by how they consistently reflect light as if polished, smooth, and even. The cleavage properties of a mineral are described in terms of the number of cleavages and, if more than one cleavage, the angles between the cleavages. The number of cleavages is the number of directions in which the mineral cleaves.
- A mineral may exhibit 100 cleavage surfaces parallel to each other. Those represent a single cleavage because the surfaces are all oriented in the same direction. The possible number of cleavages a mineral may have are 1,2,3,4, or 6. If more than 1 cleavage is present, and a device for measuring angles is not available, simply state whether the cleavages intersect at 90° or not 90°.
- To see mineral cleavage, hold the mineral up beneath a strong light and move it around, move it around some more, to see how the different sides reflect light. A cleavage direction will show up as a smooth, shiny, evenly bright sheen of light reflected by one set of parallel surfaces on the mineral.
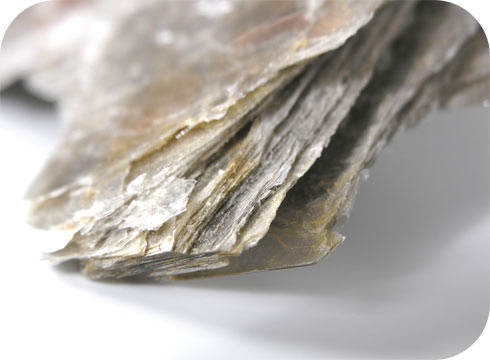
Fig. Sheets of mica
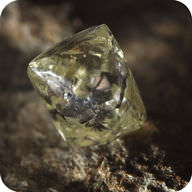
Fig. This rough diamond shows its octahedral cleavage.
Q11) Explain what is fracture and crystal shape?
A11) Fracture
- A fracture is a break in a mineral that is not along a cleavage plane. Fracture is not always the same in the same mineral because the fracture is not determined by the structure of the mineral.
- Minerals may have characteristic fractures. Metals usually fracture into jagged edges. If a mineral splinters like wood, it may be fibrous. Some minerals, such as quartz, form smooth curved surfaces when they fracture.
- Some minerals, such as quartz, have no cleavage whatsoever. When a mineral with no cleavage is broken apart by a hammer, it fractures in all directions.
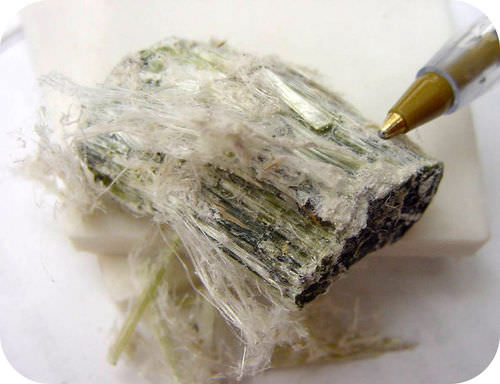
Fig. Chrysotile has a splintery fracture.
Crystal Shape
All minerals are crystalline, but only some have the opportunity to exhibit the shapes of their crystals, their crystal forms. Many minerals in an introductory geology lab do not exhibit their crystal form.
If a mineral has space while it grows, it may form natural crystals, with a crystal shape reflecting the geometry of the mineral’s internal crystal lattice. The shape of a crystal follows the symmetry of its crystal lattice. Quartz, for instance, forms six-sided crystals, showing the hexagonal symmetry of its crystal lattice.
There are two complicating factors to remember here:
(1) Minerals do not always form nice crystals when they grow, and
(2) A crystal face is different from a cleavage surface. A crystal face forms during the growth of the mineral. A cleavage surface is formed when the mineral is broken.
Q12) What is susceptibility of minerals to alternation.
A12) Susceptibility of minerals to alteration
- The description of the type and degree of alteration of coarse mineral grains, and the nature of their alteration products, is important for the interpretation of the coarse fraction of the groundmass. The term alteration is used here in a wide sense, covering changes caused both by geological processes (e.g., burial diagenesis, deuteric reactions, hydrothermal activity) and by pedogenic processes. The latter type of alteration, resulting from interaction with atmospheric agents and organisms in earth-surface conditions, is generally called weathering.
- It is evident but sometimes overlooked by micro morphologists, that changes requiring high temperatures or pressures cannot take place in soils and therefore cannot be interpreted as pedogenic.
- Susceptibility to alteration is a reflection of mineral stability in soil environments, which is determined by many factors, including temperature, moisture conditions, pH, redox conditions, degree of leaching, and the nature, composition, and grain size of the mineral. For intrinsic mineral stability, a sequence was already proposed by Goldich (1938), limited to minerals that are common in magmatic rocks.
- He proposed two parallel series, one from olivine over augite and hornblende to biotite, and the other from Ca-rich to Na-rich plagioclase, both continued by a series from potassium feldspar over muscovite to quartz. Related efforts for mineral stability classification are various weathering indexes, based on chemical composition and crystal structure.
- These approaches are not always applicable because other factors may interfere. For instance, augite may be less stable than associated olivine in basalt because of its prominent cleavage. Classifications of mineral stability that have been proposed in sedimentology are also not entirely suitable in pedology because they take physical stability during transport into account, which is not relevant for soil environments.
- Alteration of mineral grains can occur in the form of transformation to newly formed mineral phases, but it can also consist of congruent dissolution, without in situ formations of alteration products. In this case, with calcite and quartz dissolution as common examples, circum-granular voids (also called contact voids) are formed, separating the affected grain from surrounding or neighboring components. This space can later be filled with other minerals, giving the false impression of mineral transformation or coupled dissolution-precipitation.
- In these and other contexts, the formation of an enclosing mineral phase can contribute to the dissolution of the surrounded grain through the effect of crystallization pressure on solubility. After complete dissolution of a mineral grain, a mouldic void, preserving the original shape of the grain, can be left, if the surrounding material is sufficiently coherent. This has mostly been documented for minerals such as gypsum or calcite, but under tropical conditions, it can also occur for less soluble minerals such as quartz. After their development, mouldic voids can be filled with authigenic minerals, resulting in the development of pseudomorphs after the dissolved phase.
- The same result can be reached by gradual dissolution and mineral formation, which often requires an input of elements from external sources. Examples include goethite pseudomorphs after garnet, requiring Fe input, and gibbsite pseudomorphs after feldspar, requiring Al input.
- In the case of alteration through mineral transformation, a certain crystallographic relationship can exist between the original phase and its transformation product. When a mineral is completely replaced by newly formed material, by dissolution-precipitation or transformation, an ‘alteromorph’ is formed, preserving the original boundaries of the weathered mineral.
- Alteration is described as isomorphous when the alteromorph has the same shape and size as the original mineral grain, mesomorphous when the shape has been preserved but the size has changed in one or two directions, and katamorphous when the shape has been completely lost.
- Polyphase or polygenic alteration is a common phenomenon. The original mineral is in that case altered to another product, which is, in turn, transformed to another phase. For instance, hornblende in igneous rocks can be altered to biotite by late-magmatic alteration, followed by biotite transformation to vermiculite and next to kaolinite in soil conditions, resulting in kaolinite alteromorphs after hornblende.
- If the first step of non-pedogenic alteration is not complete, two associated inherited mineral phases remain side by side, which may alter subsequently following different pathways, making interpretation quite difficult. Such complex situations can only be studied using microscopic techniques. During pedoplasmation, most unstable alteromorphs are destroyed.
- Pellicular and irregular linear weathering patterns are commonly observed for minerals without well-expressed cleavage or fracture patterns (e.g., garnet, olivine), whereas parallel and cross-linear patterns point to minerals with prominent clear cleavage (e.g., pyroxenes, amphiboles, micas).
- Besides chemical weathering, also physical weathering is visible in thin sections. The most important processes are fracturing by the growth of ice or pedogenic minerals in cracks.
- The latter include salt weathering, which is mainly observed for arid environments, but similar processes can take place in tropical soils, for instance through gibbsite formation. Because the mineralogical and chemical composition of the grains does not change, this process cannot be detected by various methods, except thin section studies and grain-size analyses.
Q13) Explain what is optimal mineralogy?
A13) Optical Mineralogy
- Optical mineralogy involves studying rocks and minerals by studying their optical properties. Some of these properties are macroscopic and we can see them in mineral hand specimens. But generally, we use a petrographic microscope, also called a polarizing microscope (Figures 5.1 and 5.2 show examples), and the technique is called transmitted light microscopy or polarized light microscopy (PLM).
- A fundamental principle of PLM is that most minerals – even dark-colored minerals and others that appear opaque in hand specimens – transmit light if they are thin enough. In standard petrographic microscopes, polarized light from a source beneath the microscope stage passes through samples on the stage and then to your eye(s).
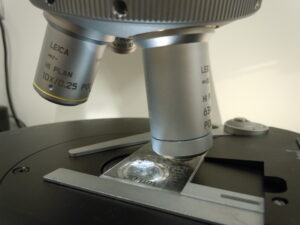
Fig. Close-up view of microscope lenses and a thin section
- One approach to PLM involves examining grain mounts, which are ground-up mineral crystals on a glass slide. The grains must be thin enough so that light can pass through them without a significant loss of intensity, usually 0.10 to 0.15 mm thick. We surround a small number of grains with a liquid called refractive index oil, and then place a thin piece of glass, called a coverslip, over the grains and liquid. The photo in Figure, below, shows garnet grains in a grain mount.
- Grain mounts and refractive index oils are necessary for making some types of measurements but are not the focus of this chapter. They were extensively used in the past, but are not much used today. For more detailed information about studying minerals in grain mounts consult an optical mineralogy
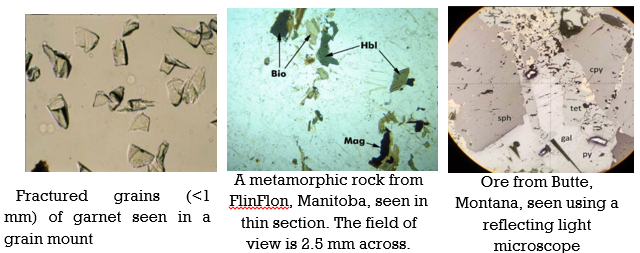
- Most optical mineralogy today involves specially prepared thin sections (0.03-mm-thick specimens of minerals or rocks mounted on glass slides).Figure 1.13 above shows a microscope view of a thin section that contains several minerals (biotite, hornblende, and magnetite are labeled, and the clear grains around them are mostly quartz and plagioclase).
- Whether looking at grain mounts or thin sections, transmitted light microscopy allows us to determine and measure properties that are otherwise not discernible. We can identify minerals, sometimes their compositions, and we can observe mineral relationships that allow us to learn about mineral origins.
- Minerals with metallic luster and a few others are termed opaque minerals. They will not transmit light even if they are thin-section thickness. So they always appear black when viewed with a microscope.
- Magnetite is an opaque mineral; the photo in Figure 1.13 contains several small black magnetite grains. For studying opaque minerals, transmitted light microscopy is of little use. Reflected light microscopy (RLM), a related technique, can reveal some of the same properties.
- As the name implies, when using RLM, the light source is above the sample and light reflects from the sample to our eye. RLM, although an important technique for economic geologists who deal with metallic ores, is not used by most mineralogists or petrologists. So we discuss it only briefly in this book. Figure 1.14 shows a view of ore from Butte, Montana, seen with a reflecting light microscope. It contains several opaque minerals: galena, sphalerite, pyrite, and chalcopyrite. They appear in various shades of black, gray, and light yellow.
- Most minerals can be identified when examined with a petrographic microscope, even if unidentifiable in hand specimens. Optical properties also allow a mineralogist to estimate the composition of some minerals. For example, we can learn the magnesium-to-iron ratio of olivine, (Mg,Fe)2SiO4, based on optical properties. And we can also determine the albite and anorthite content of plagioclase feldspar.
- Table summarizes the optical properties used for mineral identification and gives the properties of some common minerals. At the largest level, we divide minerals into opaque minerals and nonopaque minerals.
- Opaque minerals will not transmit light unless the mineral grains are much thinner than normal thin sections. We further divide nonopaque minerals into those that are isotropic (having the same properties in all directions) and those that are anisotropic (having different optical properties in different directions).
- Finally, we divide the anisotropic minerals into those that are uniaxial and those that are biaxial, and according to whether they have a positive or negative optic sign. We discuss the details of these and other diagnostic properties below.
- Besides mineral identification, the polarizing microscope reveals important information about rock-forming processes (petrogenesis). When we examine thin sections, distinguishing igneous, sedimentary, and metamorphic rocks is often easier than when we look at hand specimens.
- More significantly, we can identify minerals and distinguish among different types of igneous, sedimentary, and metamorphic rocks. The microscope allows us to see textural relationships in a specimen that give clues about when and how different minerals in an igneous rock formed. Microscopic relationships between mineral grains allow us to determine the order in which minerals crystallized from magma, and we can identify minerals produced by alteration or weathering long after the crystals first formed. Similar observations are possible for sedimentary or metamorphic rocks. Only the microscope can give us such information, information that is essential if rocks are to be used to interpret geological processes and environments.
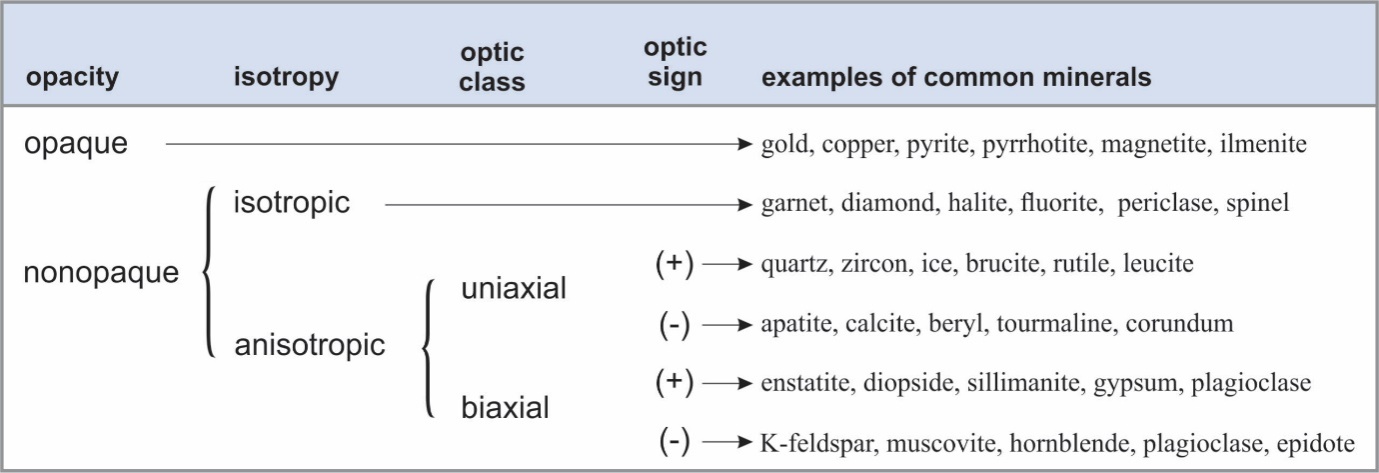