Unit - 4
Series of functions
Q1) Explain series of a function.
A1)
A series is the sum of the terms of a sequence. Thus if is a sequence then the sum
of all the terms is called an infinite series and it is denoted by

The sequence is called the sequence of partial sums of the series and the partial sums
so on, is regarded as the approximation to the full infinite sum
of the series.
If the sequence { of partial sums converges the series is termed as convergent and lim
is said to be the sum of the series.
Q2) What is the necessary condition for convergence?
A2)
Let
So that { is the sequence of partial sums.
Since the series converges, therefore the sequence also converges.
Consequently
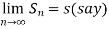
Now
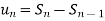
So that

Hence for a convergent series

i.e. series can not converge if its n’th term does not tend to zero.
Q3) Show that the series given below is not convergent.

A3)
Here we have

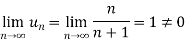
Here is not zero, therefore the given series is not convergent.
Q4) Define the continuity of a function.
A4)
A function f is said to be continuous from the right at c if
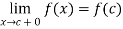
Hence a function is continuous at c if it is continuous from the left as well as from right.
Continuity at an end point- A function f defined on interval [a, b] is said to be continuous at the end point ‘a’ if it is continuous from the right at ‘a’
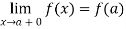
Similarly the function is continuous at the end point b of [a, b] if
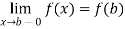
Continuity in an interval- A function f is said to be continuous in an interval [a, b] if it is continuous at every point of the interval.
Q5) A function f defined on an interval I, is continuous at a point c , and
is a sequence such that

A5)
Since f is continuous at c, so that for any given
Such that

Again since , therefore
a positive integer m, such that

From equation (1), put , we get


The sequence converges to f(c).
Or
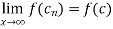
Now let f is not continuous at c.
We will now show that through a sequence
converging to c yet the sequence
does not converges to f(c).
Since f is not continuous at c, therefore there exists an
Such that for every, there exists an x such that

Also

By taking , we find that for each positive integer n, there is a
, such that

Thus the sequence does not converges to f(c).
Q6) Prove that the function defined by
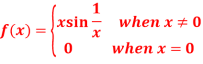
Is continuous at x = 0
A6)
Now
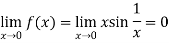
So that
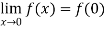
Therefore, the function is continuous at x = 0.
Q7) How many types of discontinuities are there?
A7)
Types of discontinuities-
- A function f is said to be a removable discontinuity at x = c if
exists but it is not equal to the value of f(c) of the function.
Such a discontinuity can be removed by assigning a value to the function at x = c.
2. The function f is said to have a discontinuity of the first kind at x = c if and
both exists but unequal.
3. The function f is said to have a discontinuity of the first kind from left at x = c if exists but not equal to f(c).
4. The function f is said to have a discontinuity of the second kind at x = c if neither nor
exists.
5. The function f is said to have a discontinuity of the second kind from left at x = c if does not exists
Q8) Discuss the kind of discontinuity if any, of the following function-
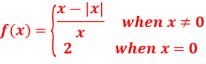
A8)
The function is continuous at all points except the origin.
Let’s test at x = 0
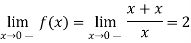
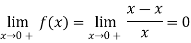
And

Thus the function has discontinuity of the first kind from the right at x = 0.
Q9) When [x] denotes the largest integer, then discuss the continuity at x = 3 for the function,

A9)
Here


And

Thus the function has a discontinuity of the first kind from the left at x = 3.
Q10) Show that the following function is continuous at origin-
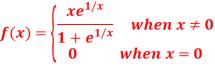
A10)
Here
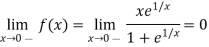
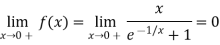
Thus

Also
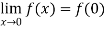
Therefore, the function is continuous at the origin.
Q11) Prove that a function which is uniformly continuous on an interval, is continuous on that interval
A11)
Suppose the function f is uniformly continuous on an interval I, so that for a given , there exists a
such that

Where are any two points of I for which |
Let , then by taking
we find that for
Such that

Hence the function is continuous at every point , which means the functions f is continuous on I.
Q12) What is the derivability in an interval?
A12)
A function defined on [a, b] is derivable at the end point a, which means f’(a) exists if
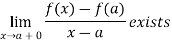
Or we can say that
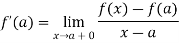
Similarly the function is derivable at the end point b, if
Or
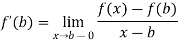
Q13) Show that the function f(x) = |x| is not derivable at x = 0.
A13)
Left hand derivative –

Right hand derivative –

Thus

Hence the function is not derivable at x = 0.
Q14) Prove that a function which is derivable at a point is necessarily continuous at that point.
A14)
Suppose the function is derivable at x = c
So that
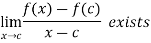
Now

Taking limits as x tends to c, we get

So that
, therefore the function f is continuous t x = c.
Q15) State and prove Weierstrass M-test.
A15)
Let < be a sequence of functions defined on E and suppose
where
is independent of x.
Then converges uniformly as well as absolutely on E if
converges.
Proof:
In order to prove uniform convergence, we see that


But since is convergent, given
, there exists N(which is independent of x) such that

Hence

And so converges uniformly by the Cauchy’s criterion for uniform convergence.
Q16) Prove that
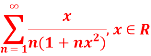
Is uniformly convergent.
A16)
We assume that x is positive, for if x is negative.
We can change the signs of all terms.
We have
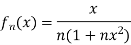
And

Thus maximum value of is
Hence

Since
is convergent, Weirstrass’s M-test implies that
is uniformly convergent for all
Q17) What do you understand by the convergence of power series?
A17)
Definition: let be a power series. Then applying Cauchy’s root test we see that the power series
is convergent if

Where

The series is divergent if

Taking
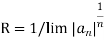
We will prove that the power series is absolutely convergent if |x| < R and divergent if |x| > R.
If are real and if x is real, we get an interval –R < x < R inside which the series is convergent.
If x is replaced by a complex number z then the power series converges absolutely at all points z inside the circle |z| = R and does not converge at any point outside the circle.
This circle is called the circle of convergence and R is known as the radius of convergence.
The interval (-R, R) is called interval of convergence.
Q18) What is the interval of convergence?
A18)
In this power series-

D’Alembert’s ratio test-

If , then by ratio test, the power series is convergent, when
is less than 1.
Or in other way if |x|<1/ then the series converges and diverges for other values.
Thus the interval of convergence of power series is-
Q19) Find the radius of convergence of the following series
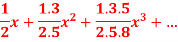
A19)
Here we have
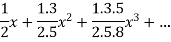
The n’th term of the series will be
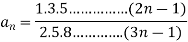
We know that
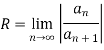
Then

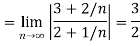
So series converges for all x where |x| < 3/2.
Q20) If the series converges, then find the value of x.
A20)
Here

Then,
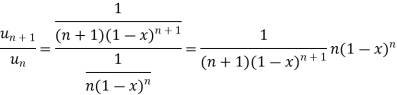

By D’Alembert’s ratio test the series is convergent for ||<1 or |1-x|>1
Or
At x = 0, the series becomes- which is divergent harmonic series.
At x = 2, the series becomes-
It is an alternate series which is convergent by Leibnitz rule.
So that the series.
Q21) State and prove Cauchy-Hadamdard theorem.
A21)
Cauchy-Hadamdard theorem- If R is the radius of convergence of the power series , then the series is absolutely convergent if |x| < R and divergent if |x| > R.
Proof:
Since the n’th term of the series is
, for each x belongs to R, let
, then
Case-1: suppose 0 < R < +
In this case,
So if |x| < R, then and hence the power series
is convergent by Cauchy root test, and
If |x| > R, then . So power series
is divergent by Cauchy root test.
Case-2: suppose R = +
Then , therefore
for each x belongs to R. Hence by Cauchy root test, power series
is convergent for each
Case-3: Suppose R = 0
In this case, , so that
for x
, therefore by Cauchy root test, the power series
is divergent for x
Q22) State and prove first form of Abel’s theorem.
A22)
Abel’s theorem (first form)
If a power series converges at the point R of the interval of convergence (-R, R), then it uniformly converges in the interval [0, R].
Proof:
Let us consider the sum

Then we have


And so on.
This gives
…..(1)
Let e > 0 be given
Now the series is convergent at x = R.
The series of numbers is convergent and hence by Cauchy’s general principal of convergence, there exists an integer N such that

Now if we take , then we have
……(3)
Consider for all
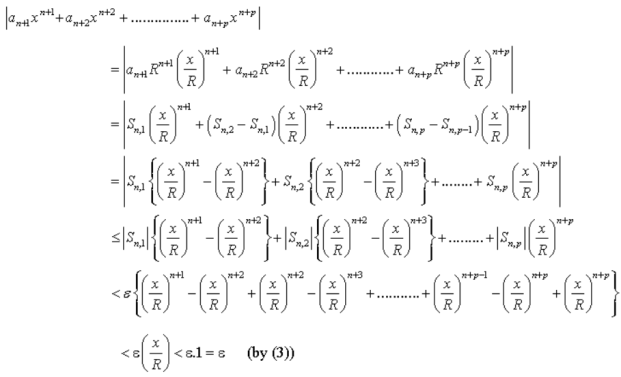
Thus we have proved

Hence by Cauchy’s criterion of convergence of series, the series converges uniformly on [0, R].
Q23) Prove that the following series is uniformly convergent.
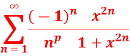
A23)
Here we note that if p > 1, then is absolutely convergent and is independent of x.
Hence by Weierstrass’s M-test, the given series is uniformly convergent for all x
If , the series
is convergent but not absolutely.
Let
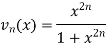
Then <> is monotonically decreasing sequence for |x| < 1, because

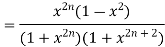
Also
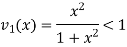
Hence, by Abel’s test, the series
Is uniformly convergent for and |x| < 1.
Q24) State and prove Weierstrass Approximation Theorem
A24)
Statement- if f is real function defined on [a, b] then there exists a sequence of real polynomials which converges uniformly to f(x) on [a, b], which means

Proof:
If a = b, then f(x) = f(a)
Then the theorem is true by taking to be a constant polynomial defined by

Thus we assume that a < b

We take a = 0, b = 1.
Now we know that for positive integer n and k where , the binomial coefficient
, which is defined as
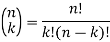
Now we define the polynomial where

The polynomial (1) is known as Bernstain polynomial.
We shall prove that certain Bernstain polynomial exists which uniformly converges to f on [0, 1].
Consider the identity

Diff w.r.t. x, we get


Multiply by x(1-x) both sides, we get

Diff. w.r.t. x, we get

On applying (2) we get

Multiply by x(1-x), we get


Since the maximum value of x(1-x) in [0, 1] is ¼.


So the equation (4) can be written as

Now f is continuous on [0,1]. So, f is bounded and uniformly continuous on [0, 1].
such that

And by uniform continuity for given

Now for any fixed but arbitrary x in [0,1], then n-values 0,1,2,3,….,n of k can be divided into two parts as follows
Let A be the set of values of k for which and B be the set of remaining values for which
Now for , we get by (5)

.
Now

By (2)
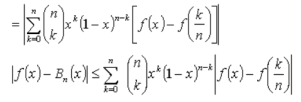
We split the summation on RHS into two parts accordingly as
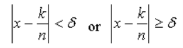
Let
Thus we have
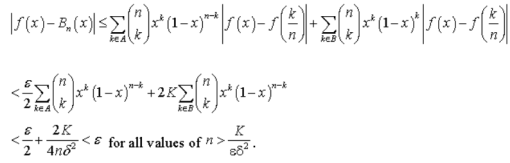
Thus converges uniformly to f(x) on [0, 1].
Which is the proof of the theorem.