Unit - 1
Basics of Organic Chemistry
Q1) Explain the Inductive effect?
A1) Inductive Effect occurs in a molecule, in which the bonding electrons are unequally shared resulting in the formation of a permanent dipole. The Inductive effect occurs in sigma bonds while the electrometric effect arises in pi bonds.
The Inductive Effect
The inductive effect, sometimes written as "the -I Effect" in literature, is the distance-dependent phenomenon by which the charge of a chemical bond affects orientation on adjacent bonds in a molecule, producing a permanent state of polarization.
The electron density of a σ bond is not uniform when atoms of two different elements participate in the bond. Electron cloud in a bond tend to orient themselves toward the more electronegative atom involved in the bond.
The inductive effect can be effectively seen in water molecules, the chemical bonds present in water molecules are more positively charged at the hydrogen atoms and more negatively charged near the oxygen atom. Therefore, the water molecules are polar in nature. Note, however, the induced charge is weak and the inductive effect is only active across short distances, so other factors can quickly overcome it.
Inductive Effect and Acidity and Basicity
The inductive effect affects the stability as well as acidity or basicity of a chemical species. Electronegative atoms draw electrons toward themselves, which can stabilize a conjugate base. Groups that have -I effect on a molecule decrease its electron density, making the molecule electron deficient and more acidic.
Types of Inductive Effect
- Negative inductive effect or -I effect
- Positive inductive effect +I effect
-I Effect (Negative Inductive Effect)
When an electronegative atom , such as a halogen, is introduced to a chain of atoms (generally carbon atoms), the resulting unequal sharing of electrons generates a positive charge which is transmitted through the chain.
This causes a permanent dipole to arise in the molecule wherein the electronegative atom holds a negative charge and the corresponding effect is called the electron-withdrawing inductive effect, or the -I effect.
+I Effect (Positive Inductive Effect)
When a chemical species with the tendency to release or donate electrons, such as an alkyl group, is introduced to a carbon chain, the charge is relayed through the chain and this effect is called the Positive Inductive Effect or the +I Effect
Inductive Effect on Stability of Molecules
The charge on a given atom and the charge on a group bonded to the atom play a strong part when determining the stability of the resulting molecule as per the inductive effect.
An example of this can be observed when a group displaying the -I effect is bonded to a positively charged atom and the positive charge on the resulting molecule is amplified, reducing its stability.
On the other hand, when a negatively charged, atom is introduced to a group displaying a -I effect, the charge disparity is somewhat quenched and the resulting molecule would be stable as per the inductive effect.
Application of Inductive Effect
- Stability of carbocation
- Stability of carbanion
- Stability of free radicals
- The acidity of carboxylic acid
- Basicity of amines
Q2) What is the importance of the Resonance effect?
A2) The single dot Lewis structure, cannot be used to predict the properties of many organic compounds, when we take the example of benzene, if we consider the Lewis dot structure, it results show Benzene to have three C-C bonds and three C=C bonds. But, the actual property deviates from this prediction.
Thus, Resonance is applied to define the properties of such compounds, The resonance structures (canonical structures) are actually hypothetical. This is because they do not represent any real molecule individually. They contribute to the actual structure in proportionately according to their stability.
The energy of actual structure of the molecule (the resonance hybrid) is lower than that of any of the canonical structures. The resonance energy increases with the number of important contributing structures. The number of unpaired electrons is the same in the resonance structures and so also are the positions of nuclei.
Stability
The stability of resonance increases with:
- Number of covalent bonds
- Number of atoms with an octet of electrons (except hydrogen which has a duplex)
- Separation of opposite charges,
- Dispersal of charge
- A negative charge if any on a more electronegative atom, a positive charge if any on the more electropositive atom, increases the stability of the atom.
More on Resonance Effect
Resonance is the phenomenon which causes a polarity to be produced in the molecule. This could happen either by the interaction of two π-bonds or between a π-bond and lone pair of electrons present on an adjacent atom. The delocalisation of π-electrons is what causes this effect. We can classify the resonance effect into two main categories, as described below.
1) Positive Resonance Effect (+R effect)
In the positive resonance effect, we notice that the transfer of electrons takes place away from an atom or substituent group attached to the conjugated system (presence of alternate single and double bonds in an open-chain or cyclic system) due to resonance. Some of the substituent groups which attribute to positive resonance effect are – COOH, –CHO, >C=O, – CN, –NO2, etc.
2) Negative Resonance Effect (-R effect)
In this effect, we see that the transfer of electrons is towards the atom or substituent group attached to the conjugated system (presence of alternate single and double bonds in an open-chain or cyclic system) due to resonance. Examples of the substituent groups that attribute to negative resonance effect include – COOH, –CHO, >C=O, – CN, –NO2, etc.
Q3) Explain Hyper conjugation?
A3) The hyperconjugation is a permanent effect, that involves the localization of the of σ electrons of C-H bond connected to an alkyl group directly attached to an atom of the unsaturated system or to an atom with an unshared p orbital takes place.
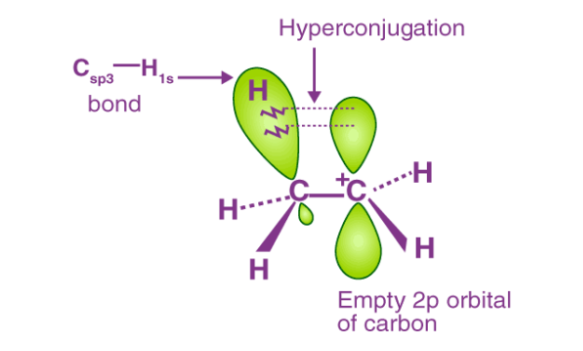
Fig: From the diagram, on observation the three C-H bonds of the methyl group can align itself in the plane of the empty p orbital and the electrons constituting the C-H bond in a plane with this p orbital can then be delocalized into the empty p orbital.
During hyperconjugation, the carbocation is stabilized as the dispersal of the positive charges is maintained, thus it is said that greater the number of alkyl groups attached to a positively charged carbon atom, the greater is the hyperconjugation interaction and stabilization of the carbonation. The relative stability on the basis of hyperconjugation is given as,
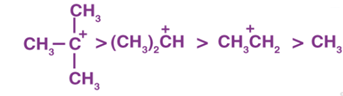
Causes of hyperconjugation
Hyperconjugation is described as the stabilizing interaction, results from the interaction of the electron in a pi bond (in general, C-C or C-H) with either an adjacent filled or empty p-orbital or a pi-orbital to form an extended molecular orbital, which increases the stability of the system.
Depending on the bonding valence bond model, hyperconjugation is described as the ‘double bond”-no bond resonance. It is not what we would “generally” call resonance, though the similarity is perhaps useful.
Applications of hyperconjugation
(1) Stability of alkenes: the stability of few alkenes over the other ones are explained by hyperconjugation.
Stability of alkenes ∝ Number of alpha hydrogens ∝ Number of resonating structures

Stability in decreasing order
(2) Carbon-carbon double bond size in alkenes: the more the number of resonating structures, the more will be single bond character in carbon-carbon double bond.
(3) Durability of alkyl carbocations: Stability of alkyl carbocations ∝ number of resonating structures µ number of alpha hydrogens.
(4) Stability of alkyl free radicals: the stability that is present alkyl free radicals is explained by hyperconjugation, the number of resonating structures determines the stability.
(5) Electron releasing (or donating) power of R in alkyl benzene: CH3- (or alkyl group) is +R group, ortho-para directing set and activating set for electrophilic aromatic substitution reaction because of the hyperconjugation.
The electron donating power of alkyl group will depends on the number of resonating structures, this relays on the number of hydrogens present on a-carbon. The electron releasing power of some groups are as follows,
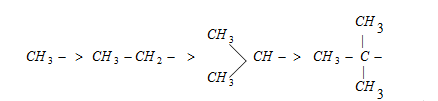
Increasing inductive effect
Electron donating power in descending order due to the hyperconjugation.
(6) Heat of hydrogenation: the heat of hydrogenation is decreased by Hyperconjugation.
(7) Dipole moment: Since hyperconjugation affects the development of charges, it also affects the dipole moment in the molecule.
The increase in dipole moment, when hydrogen of formaldehyde (μ = 2.72D) is replaced by methyl group, i.e., acetaldehyde (μ = 2.72D) can be tends to hyperconjugation, which tends to development of charges.
(8) Orienting influence of alkyl group in o, p-positions and of -CCl3 group in m-position: Ortho-para directing property of methyl group in toluene is partly due to effect and partly due to hyperconjugation.
Q4) What do you understand by Dipole moment?
A4) The separation of charges in any system leads to dipole moment. Both ionic and covalently bonded compounds develop dipole moment. The main cause for the development of dipole moment is the electronegativity difference between chemically bonded atoms or elements a.
Polar Character is the separation of positive and negative charges, in a compound This measurement of polar character of a chemical bond in a molecule, between two atoms, is given by Bond dipole moment is considered as a vector Quantity, as it has both magnitude and direction. For example
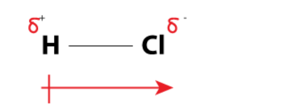
Fig: Dipole moment in HCl
δ+ and δ- indicate positive and neagtive charges that are separated by distance d, these charges are equal in magnitude but opposite in sign.
Important points
Bond dipole moment differs from the total dipole moment in polyatomic molecules e
Bond dipole moment is the dipole moment between the single bond of a diatomic molecule, while the total dipole moment in a polyatomic molecule is the vector sum of all the bond dipoles.
Thus, total molecular dipole moment depends on the factors like-difference in size of the two atoms, hybridization of the orbitals,direction of lone pair electrons.
Dipole moment can also be zero when opposite two bond dipoles cancel eachother
In chemistry the representation of dipole moment is given little differently with arrow symbol. Dipole moment is represented by an arrow with (+) on one side . The arroe side denotes the shifting of electron density of the molecule.
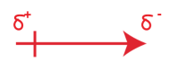
Fig: Representation of Dipole moment
Dipole moment formula
Dipole moment definition can be given as the product of magnitude of electronic charge of the molecule and the internuclear distance between the atoms in a molecule , it is given by the equation
Diploe moment (µ) = Charge (Q) x Distance of separation(d)
(µ) = (Q) x (d), where µ is the dipole moment , Q is the magnitude of the partial charges δ+ and δ- and is the distance betwenn δ+ andδ-
It is measured in Debye units, represented by D.
D= 3.33564x 10-30Cm ; C=Coulomb, m=meter.
Uses of Dipole moment
To find the polar nature of the bond : as the magnitude of dipole moment increases, more will be the polar nature of the bond. Molecules with zero dipole moment are non-polar, while molecules with dipole moment are said to be polar
To find the structure or shape of the molecules: Molecules having two or more polar bonds would not be symmetrical and possess some dipole moment, examples: H2O = 1.84D; CH3CL ( methyl chloride) = 1.86D, if similar atoms in the molecule are attached to the central atom with resultant dipole moment zero, then such molecules will have symmetrical structures, Examples: CO2,CH4
Q5) Explain the significance of the Mesomeric effect?
A5) The Mesomeric effect was developed by a scientist Ingold in 1938, it’s also known as themesomer or the mesomerism, it’s actually a synonym to resonance which was discovered by scientist Pauling.
The word mesomerism was extensively used in the French and German language till 1950. But in English language, resonance gained prominence and is widely used today, over all it refers to the same product.
What is Mesomeric Effect?
Mesomeric effect refers to the polarity that occurs between the atoms of a conjugated system by the transfer of electrons or the transfer of pi electrons, putting it in a simple way the mesomeric effect develops when pi electrons move away from or towards a substituent group in a conjugated orbital system.
The mesomeric effect can be subdivided into two types:
- +M effect
- -M effect
+M effect (Positive mesomeric effect)
When the electrons or the pi electrons are transferred from a particular group towards a conjugate system, thus increasing the electron density of the conjugated system then such a phenomenon is known as (+M) effect or positive mesomeric effect.
Example 1:
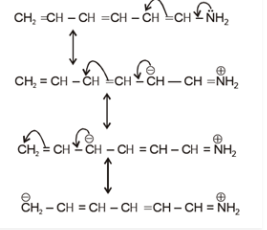
Example 2:
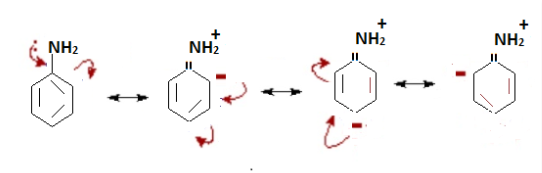
- For the +M effect, the group should have either a lone pair of electrons or should have a negative charge.
- The +M effect gives negative charge to the conjugate system or it can be said that the electron density increases on the conjugate system due to this. These conjugate systems show more reactivity towards electrophiles and less reactivity towards a nucleophile.
Group showing +M effect
–NH, –NH2,–NHR, –NR2, – O, – OH, –OR, – F, – Cl, –O–COR, – NHCOR, –SH, – SR etc.
-M Effect (Negative mesomeric effect)
When the pi-bond electrons are transferred from the conjugate system to a particular group thus the electron density of the conjugate system is decreased, then this phenomenon is known as negative mesomeric (–M) effect.
- For –M effect, the group should have either a positive charge or should have a vacant orbital.
- –M effect makes the compound more reactive towards a nucleophile as it decreases the electron density in the conjugate system and at the same time it is less reactive towards electrophile due to same reasons.
The group which shows –M effect include;
–NO2, –CN, –COX, –SO3H, – CHO, –CONH2, –COR, –COOH, –COOR etc.
Significance of Mesomeric effect
- It describes the distribution of the charge in the compound, helps to decide the point at which electrophiles or nucleophiles attack.
- Useful in describing physical characteristics such as dipole moment, bond length.
Q6) Write a note on Organic Acids and Bases?
A6) The theory on Acids and bases was first postulated by Arrhenius, the theory states that the acids dissociate in water to form protons(H+) and the bases dissociate in water to form (–OH):
Arhrenius Acids and Bases
Acids dissociate in water to form protons (H+)
Bases dissociate in water to form (-OH)
HCI -> H + + Cl –
NaOH -> Na + - OH
Restrictions: 1) only in water 2) only – OH is a base.
The organic bases do not react with acids; hence this theory has limitations, but are not hydroxide ions.
Hence the Brønsted-Lowry acids and base theory came into being, and is effectively used to qualify compounds as acids or bases. According to this theory it is considered more universal than the Arrhenius theory and it gives details that bases as species that are proton acceptors which include the hydroxide ions but are not limited to them:
Bronsted Acids and Bases:
Acids are proton donors
Bases are proton acceptors
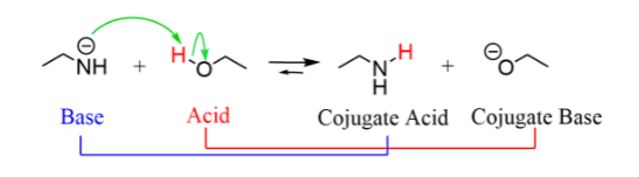
Fig: The movement of electrons in the Bronsted Acids and base reactions
When we consider proton transfer in acid-base reactions, like in many reactions but the curved arrows, like for any other reaction, show the movement of electrons.
In a Brønsted-Lowry acid-base reactions, the base turns into its conjugate acid and the acid turns into its conjugate base.
Their relative strength
The strength of a Brønsted-Lowry acid or base depends on the extent to which it ionizes in water. Although there are numerous solvents besides water, chemists discuss acid and base strength in relation to water because they use it so widely as a solvent. Chemists use the autoionization of pure water to determine the values for the concentrations of acidic and basic solutions. Autoionization is the reaction of two molecules of water with each other to give a hydronium ion, H3O⊕, and a hydroxide ion, c- OH.

For this reaction, the amount of autoionization is extremely slight—at 25oC, it is 10–7 M (moles/liter). The concentrations of H3O⊕ and c- OH are equal; that is, both measure 10–7 M. Chemists call this a neutral solution. If you add a compound that is more acidic than water, you increase the concentration of H3O⊕ ions and make the solution acidic. If you add a compound that is more basic than water, you increase the concentration of c- OH ions and make the solution basic. The product of the H3O⊕ and c- OH concentrations in water is equal to 10–14 and is a constant, Kw. Chemists define Kw with the following equation.

Because the concentrations of H3O⊕ and c- OH are equal in a neutral solution, you can easily calculate the concentration of both: When performing a concentration calculation, replace the chemical species listed within the brackets, [c- OH], for example, with that species' molar concentration.

As the concentrations of both the products is a constant it is denoted by Kw, when the concentration of one product increases the concentration of the other will must decrease, as the concentration of both the product is a constant. For example, if C-OH ions are added to water, the H3O ions concentration decreases, by whatever amount is necessary for the product of the two concentrations to still equal 10–14. Because the hydronium ion concentrations can span a very wide range of values, from greater than 1 M down to less than 10–14 M, chemists measure the concentration of H3O⊕ on a logarithmic scale called pH. The values of pH produce the hydronium ion concentration of a solution; therefore, the pH of a solution is a mode of quantifying the acidity of that solution.
Chemists define measurement as the negative logarithm (base 10) of the H3O⊕ concentration, represented by the following equation:

For simplicity, this book will normally refer to the H3O⊕ ion as the H⊕ ion from now on. If an equation shows the H⊕ ion present in aqueous solution, remember that it is actually the H3O⊕ ion. This equation shows the general reaction of an acid in water:
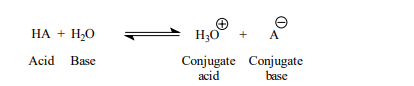
A Conjugate acid Conjugate base Acid Base, this equation is in equilibrium, as a matter of fact most of the acid-base reactions are in equilibrium as the reactant ionize partly. Strong acids and bases ionize completely in water. Weak acids and bases ionize only partially in water.
A solution is said to be acidic when the aqueous solution has a concentration of hydrogen ions greater than 10-7 M, similarly a solution is said to be basic when the concentration of the hydroxide ions is greater than 10-7M. To determine the relative strength of an acid or a base, you need to find out how much the acid or the base ionizes, or dissociates, in water at equilibrium. The equilibrium constant, Ke, gives this information and is defined as follows:
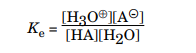
However, because water is the solvent and its concentration is essentially constant, a more meaningful value for acid ionization comes from multiplying the equilibrium constant by the water concentration:
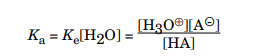
Chemists call Ka the acid dissociation constant. The Ka constant specifies the strength of the acid, The stronger the acid, the larger the amount of dissociation and the larger the concentration of H3O⊕ ions. Thus, the value of Ka will be large if the acid is stronger. Strong acids completely dissociate in water and have large dissociation constants. Most of the organic compounds have the dissociation constant that range from 10–2 to 10–60. Because acids have such a large range of values for their dissociation constants, they are often convert those values to a logarithmic scale, similar to pH. The following equation defines this scale:

Q7) Describe the Homolytic fission?
A7) The process or the manner, where the chemical bond breaks play a crucial role in the overall chemical reaction. The breakage of a chemical bond (usually a covalent bond) is often referred to as bond fission. The two primary types of bond fission are homolytic fission and heterolytic fission.
Homolytic Fission
Homolytic fission (sometimes referred to as haemolysis) is a type of bond fission that comprises the dissociation of a molecule, where one electron is retained by each of the original fragments of the given molecule. Therefore, when a neutrally charged, molecule is subjected to homolytic fission, two free radicals are obtained as the product (since each of the chemical species retains one electron from the bond pair).
The homolytic fission is also called homolytic cleavage or bond analysis. And the term is derived from Greek word for “homo” meaning root and the term roughly meaning “equal breaking”
The energy required to facilitate homolytic fission in a molecule is often referred to as the homolytic bond dissociation energy of the molecule. An illustration detailing the homolytic fission of a molecule AB, resulting in the formation of two free radicals (Ao and Bo) is provided below.

Fig: formation of two free radicals
Typically, a large amount of energy is required to spark the homolytic fission of a molecule. This is the reason why this type of bond fission only occurs in some cases, as listed below.
- When the molecule is subjected to ultraviolet radiation (the electromagnetic radiation corresponding to the ultraviolet region of the electromagnetic spectrum)
- When the molecule is subjected to the required amount of heat in order to overcome the required bond dissociation energy for the homolytic fission
- When carbon compounds are subjected to extremely high temperatures in the absence of oxygen in order to facilitate the pyrolysis of the molecule
In some cases, homolytic fission can be achieved by supplying only a small amount of heat to the molecule. One such example is the homolytic cleavage of the oxygen-oxygen bonds in peroxides. These intramolecular bonds are fairly weak, implying that they have very small bond dissociation energies. Therefore, this barrier can be overcome with only a small amount of heat energy.
Q8) Highlight the curly arrow rules?
A8) The curly arrows are used to show the single movement of electrons these arrows are only used to show the movement of single electrons. Similar arrows can be used in case pf pair of electrons also, in case of single electrons the head of the arrow have a single line rather than two lines. The picture below shows the movement of an electron pair, shows the movement of electron for a single pair, the first stage of polymerisation of ethene is shown below:

The dots can be drawn to show the specified electrons, the half arrow indicates the movement of these dots, care should be taken to indicate the arrow as half arrows or else the whole meaning of the reaction and the meaning of the single pair is lost. Are some cases the syllabus can indicate to just ignore the arrow in such cases the half arrows can be avoided for a reaction.
The curly arrows are used in mechanisms to show the various electrons moving around, in any case they must not be used for any other purpose.
- The arrow tail is where the electron pair starts from. But we have to write about the electron pair either as a bond or a lone pair of electrons, as a pair of dots, lone pair of electrons are electrons at the bonding level.
- The arrow head is where the electron pair to end up.
For example, when we take the example of a reaction between ethene and hydrogen bromide, one of the two bonds between the two carbon atoms breaks
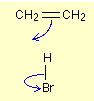
Those electrons move to form a new bond with the Hydrogen from HBr at the same time the pair of electrons move toward the Br atom from HBr.
There is no need to draw the pair of electrons in the bonds as two dots, drawing the bond as a line is sufficient, notice that the arrow head points between C and H because that’s where the electron pair ends up, the electron movement between H and Br is shown down as a curly arrow even though the pair moves straight down, the electron pair movements should be shown as curly arrows,
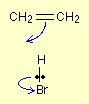
The second stage of this reaction shows a good illustration on how curly arrows are used if a lone pair of electrons is involved.
The first stage leaves you with a positive charge on the right-hand carbon atom and a negative bromide ion.
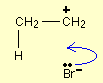
The lone pair on the bromide ion moves to form a new bond between the bromine and the right-hand carbon atom. That movement is again shown by a curly arrow. Notice again, that the curly arrow points between the carbon and the bromine because that's where the electron pair ends up.
That leaves you with the product of this reaction, bromoethane:
Q9) Explain the Heterolytic Fission?
A9) Heterolytic fission, also known as heterolysis, is a type of bond fission in which a covalent bond between two chemical species is broken in an unequal manner, resulting in the bond pair of electrons being retained by one of the chemical species (while the other species does not retain any of the electrons from the bond pair). When a neutrally charged molecule undergoes heterolytic fission, one of the products will have a positive charge whereas the other product will have a negative charge.
The positive charge product of the heterolytic fission of a neutral molecule is usually called as the cation, it is the chemical species, it is the chemical species that does not retain the bonded electrons after the bond fission, On the other hand, the negatively charged product of the heterolysis (also known as the anion) is the chemical species that retains both the bonded electrons after the bond fission process.
The term ‘heterolysis’ has Greek roots and can be roughly translated as ‘unequal breaking’. It is also referred to as homolytic cleavage. An illustration detailing the two ways in which a molecule AB can undergo heterolytic fission is provided below. In the first scenario, the bond pair of electrons is retained by B, making it the anion and A the cation. In the second scenario, A retains the bond pair and becomes the anion whereas B becomes the cation.
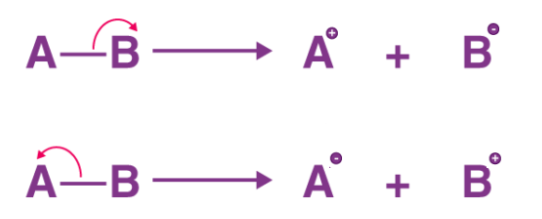
Fig: Example of heterolytic Fission
It can also be noted that when a covalent bond is subjected to heterolytic fission, the bonded species with the greater electronegativity is the one that usually retains the bond pair of electrons and obtains a negative charge. On the other hand, the more electropositive species usually does not retain any electrons and obtains a positive charge.
The energy required to cleave a covalent bond via heterolytic cleavage is often referred to as the heterolytic bond dissociation energy (not to be confused with homolytic bond dissociation energy). This value is sometimes used to denote the bond energy of a covalent bond. An example of homolytic fission can be observed in the hydrogen chloride molecule, as illustrated in the chemical reaction provided below.
H-Cl → H+ + Cl–
Here, the chlorine atom retains the bond pair of electrons because its electronegativity is higher than that of hydrogen. Therefore, the products formed are the chloride anion and the hydrogen cation.
Q10) Differentiate between Electrophiles and Nucleophiles?
A10) Electrophiles and Nucleophiles
Electrophile and nucleophile are the species that either accept or donate electrons to form a new chemical bond, the Nucleophile is a chemical species on response gives an electron pair to form a chemical bond, any ion or atom or molecule that in some way is deficient in electron acts as an electrophile.
A nucleophile is usually negatively charged or it may also be neutral consisting of a lone pair of doable electrons. H2O, -OMe or -OtBu are some examples. On the whole the electron rich species is a nucleophile and Electrophiles are positively charged or may be neutral and consists of empty orbitals that attracts a range of electrons.
The chemical reactions that occur between the electron acceptors and donors are described by the concepts like electrophiles and nucleophiles.
Electrophile
Positively loaded or neutral species are called electrophiles that are deficient in electrons and can accept a couple of electrons. These are also called species that love electron (philic).
- The term electrophile can be split into “electro” derived from electron and “phile” which means loving.
- They are electron deficient and hence electrons loving.
- They are positively charged or neutrally charged.
- They attract electrons. Movement of electrons depends on the density.
- They move from high-density area to low density area.
- They undergo electrophilic addition and electrophilic substitution reactions.
- An electrophile is also called as Lewis’s acid.
Nucleophile
A nucleophile is a reagent or a chemical species that consists of an unparallel or lone pair of electrons, they are wealthy in terms of electrons, they seek for locations that are electron deficient. i.e., nucleus means loving nucleus. Nucleophiles act as Lewis bases, according to Lewis ‘notion of acids and bases.
- The term nucleophile can be split into “nucleo” derived from the nucleus and “phile” which means loving.
- They are electron rich and hence nucleus loving. They are negatively charged or neutrally charged.
- They donate electrons.
- Movement of electrons depends on the density.
- They move from low-density area to high-density area.
- They undergo nucleophilic addition and nucleophilic substitution reactions.
- A nucleophile is also called as Lewis base.
For example, as nitrogen is less electronegative than oxygen, ammonia is a strong nucleophile than water. This means that the loosely bound nitrogen lone pair of ammonia is stronger than the oxygen bound solitary water pairs.
Difference between Electrophile and Nucleophile
The difference between Electrophile and Nucleophile is listed below. | |
ELECTROPHILE | NUCLEOPHILE |
Also called as Lewis acid | Also called as Lewis base |
They are positively charged / neutral | They are negatively charged / neutral |
They undergo electrophilic addition and electrophilic substitution reactions | They undergo nucleophilic addition and nucleophilic substitution reactions |
Electron-deficient | Electron-rich |
It accepts a pair of an electron to form a covalent bond | It donates a pair of an electron to form a covalent bond |
All carbocations | All carbanions |
Example: Hydronium Ion | Example: Chloride Ion |
Q11) Define Basicity?
A11) Basicity is the capability of the chemical species to act as a base, they are defined in various ways by the scientists. According to Arrhenius a base is a substance that donates OH– ions to the solution. Bronsted- Lowry defines a base as a substance that can accept a proton. According to Lewis, any electron donor is a base. According to the Arrhenius definition, for a compound to be a base they should have a hydroxide anion and also the ability to donate it as a hydroxide ion. But according to Lewis and Bronsted- Lowry, molecules can still be base even if they do not possess hydroxides and can still act as a base. For example, NH3 is a Lewis base, because it can donate the electron pair on nitrogen. Na2CO3 is a Bronsted- Lowry base without hydroxide groups, but has the ability to accept hydrogens.
Bases are known to have a bitter taste and a slippery soap feeling, they easily react with acids to produce water and salt molecules. Caustic soda, baking soda and ammonia are the common bases. Bases are divided into two categories the strong bases that completely ionize in a solution producing ions.e. g NaOH and KOH, the weak bases on the other hand like Ammonia are partially
Q12) Write a note on Carbocations?
A12) A carbocation is a molecule, wherein the carbon atom has a positive charge along with three bonds, they are basically called as carbon cations. They were initially called as carbonium ion, Carbocation today is defined as any even-electron cation that possesses a significant positive charge on the carbon atom.
In general, the carbo cation is very reactive and unstable as they possess an incomplete octet, in simple terms they do not have eight electrons and do not imply to the octet rule.
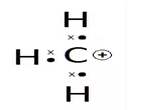
In carbocation, the hybridisation of carbon will be sp2 and its shape is trigonal planar. There is also a vacant p orbital which indicates its electron-deficient nature. The carbon has 6 electrons in its valence shell. Due to this, it is an electron-deficient species, also known as an electrophile.
A carbocation is generally observed in an SN1 reaction, elimination reaction, etc.
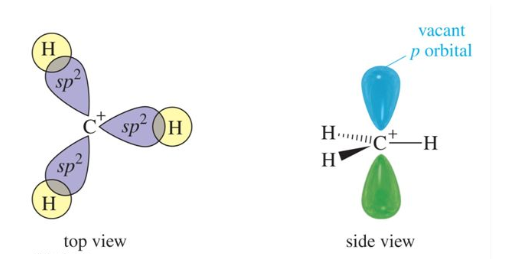
Fig: Structure of a Carbocation
Classification of Carbocation
The different carbocations are named on the basis of the number of carbon groups bonded to the carbon. The carbocation can be termed as methyl, primary, secondary or tertiary on the basis of how many carbon atoms are attached to it:
- Methyl carbocation: If no carbon is attached to the carbon with the positive charge, it is simply called as methyl carbocation.
- If one, two or three carbon is attached to the carbon with the positive charge it is called the primary carbocation, secondary carbocation, tertiary carbocation respectively.
- If there is a carbon-carbon double bond next to the carbon with the positive charge it is termed as allylic carbocation.
- In the same way, if the carbon with the positive charge is attached to a double bond, the carbocation is termed as vinylic carbocation. Here, hybridization of the carbon having the positive charge is spa and geometry is linear.
- Whenever the carbon which consists of the positive charge is part of a benzene ring, then the carbocation an aryl carbocation.
- If the carbon having a positive charge is immediately next to a benzene ring, it is termed as a benzylic carbocation.
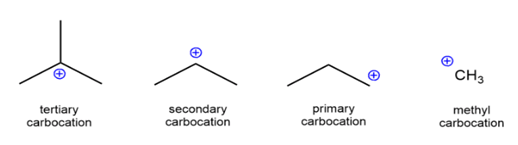
Interestingly, in addition to these types, there is another type of carbocation which is known as pyramidal carbocation. In this type, the ions consist of a single carbon atom that usually tends to hover over a four- or five-sided polygon which can be depicted as a pyramid. The 4-sided pyramidal ion will consist of +1 charge while the five-sided pyramid will have +2 charge.
Formation of the Carbocation
The carbocations can be formed by either of the following two fundamental steps:
- Cleavage of bond of carbon.
- Electrophilic addition.
Cleavage of Bond of Carbon
Whenever there is cleavage of the bond of carbon and atoms attached to it, the leaving group takes away the shared electrons. Thus leaving the carbon atom as electron deficient. As a result, a positive charge is developed forming a carbocation. The more tendency of cleavage of bond or formation of a more stable carbocation the lower is the activation energy
In many organic reactions such as the SN1 and E1 reactions carbocation is formed as a reaction intermediate.
Electrophilic Addition
In electrophilic addition, an electrophile attacks on unsaturated point (double or triple bond), this results in the breaking of the pi bond which results in the formation of a carbocation. The more stable is the carbocations the lower is the activation energy and faster addition. Electrophilic addition to a pi bond is illustrated by the reaction of HBr (an electrophile) with propene (CH3CH = CH2).
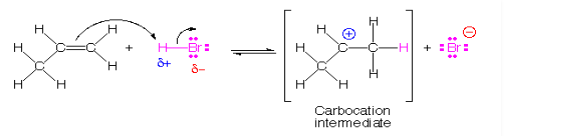
It can be noted that the formation of the secondary carbocation is favoured over the primary carbocation because secondary carbocation is more stabilized due to resonance. This is also in accordance with Markovnikov’s Rule. Such electrophilic addition reactions are generally seen in alkenes, alkynes and benzene rings.
Carbocation Stability
The stability order of carbocation is as follows:
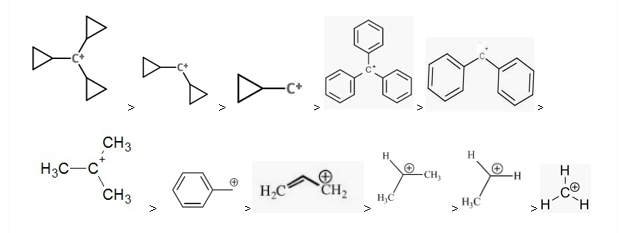
The stability of carbocations depends on the following factors:
1. Resonance: Stability of carbocations increases with the increasing number of resonances. More the number of resonating structures more is the stability of the carbocation. The reason for this is the delocalization of the positive charge. The electron deficiency is decreased due to the delocalization and thus it increases the stability.
When compared to substitution, the resonance effect proves to be a more dominating factor than substitution. Therefore, structures with resonance are far better stabilised than others. Cyclopropane carbocation is exceptionally very stable due to dancing resonance. Thus, tricyclo propane carbocation is the most stable carbocation.
2. Hyperconjugation and inductive effect: Increasing substitution, increases the hyperconjugation and thus it increases stability. More the hyperconjugation more is the stability.
R3C+ (3o ; most stable) > R2CH+ (2o ) > RCH2+ (1o) CH3+ (methyl; least stable)
The Carbocation stability depends on the number of carbon groups attached to the carbon carrying the positive charge.
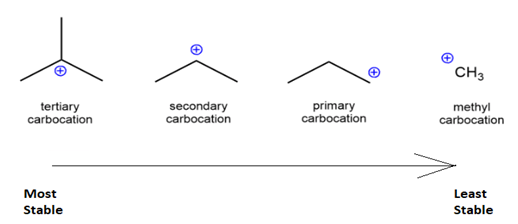
3. Electronegativity: Electronegativity indicates the capacity of an atom to attract electrons. The more is the electronegativity; the more is the attraction of the electrons towards the atom. Therefore, the electronegativity of the carbon with the positive directly affects the stability of the carbocation. So as the electronegativity of the carbon atom increases the stability of the carbocation decreases. Sp > sp2 > sp3 ( sp has maximum s character; so maximum electronegativity, sp3 has minimum s character; so minimum electronegativity).
The hybridisation of the carbon with the positive charge in the vinylic carbocation is sp whose electronegativity is more than the sp2 hybridized carbon of the alkyl carbocation. Due to this reason, the stability of a primary vinylic carbocation is less than a primary alkyl carbocation.
In the same way, lower stability of aryl carbocation in comparison to a secondary alkyl carbocation can be explained. Vinyl and aryl carbocations are very rare to find due to their low stability
Q13) Define Carbenes?
A13) Carbene is a molecule that consists of a neutral carbon atom having a valence of two and also possess two unshared valence electrons. The general formula is R-(C:)-R' or R=C:. The term "carbene" may also refer to the specific compound H2C: also called methylene, it forms the parent hydride forming the base of all carbene compounds that are formally derived.
Carbenes are classified as either singlets or triplets depending upon their electronic structure. Most of the carbenes are very short lived, although persistent carbenes are known. One well studied carbene is Cl2C:, or dichlorocarbene, which can be generated in situ from chloroform and a strong base.
Singlet and triplet carbenes
The two classes of carbenes are singlet and triplet carbenes. Singlet carbenes are spin-paired. In singlet carbenes, the molecules adopt a sp2 hybrid structure mainly in the language of the valence band theory. Triplet carbenes have two unpaired electrons. They may be either linear or bent, i.e., sp or sp2 hybridized, respectively. Most carbenes have a nonlinear triplet ground state, except for those with nitrogen, oxygen, or sulfur atoms, and halides directly bonded to the divalent carbon.
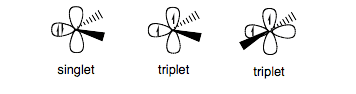
Figure: Types of Carbenes
Carbenes are classified into singlet or triplet carbenes depending on the electronic spins they acquire. The triplet carbenes are paramagnetic in nature and if they persist long enough, they can be detected by electron spin resonance spectroscopy. The total spin of singlet carbenes is zero while that of triplet carbenes is one (in units of [\hbar] ). The Bond angles range between 125-140° for triplet methylene and 102° for singlet methylene (as determined by EPR). Triplet carbenes are generally stable in the gaseous state, while singlet carbenes occur more often in aqueous media.
For simple hydrocarbons, triplet carbenes usually are 8 kcal/mol (33 kJ /mol) more stable than singlet carbenes. The stabilization is in part attributed to Hund’s rule of maximum multiplicity.
Strategies for stabilizing triplet carbenes are elusive. A rapid equilibrium mixture of the singlet and triplet states having approximately 1.1 kcal/mol (4.6 kJ/mol) energy difference is seen in a carbene called 9-fluorenylidene. It is, however, debatable whether diaryl carbenes such as the fluorene carbene are true carbenes because the electrons can delocalize to such an extent that they become in fact biradicals. In silico experiments suggest that triplet carbenes can be thermodynamically stabilized with electropositive heteroatoms such as in silyl and silyloxy carbenes, especially trifluorosilyl carbenes.
Q14) What are Free Radicals?
A14) A free radical may be defined as an atom or a group having an odd or unpaired electron. These are generally produced by homolytic cleavage of a covalent bond
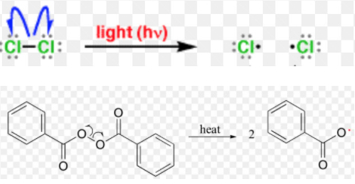
Classification of free Radicals
Free radicals are also classified as primary (1o), secondary (2o) and tertiary ( 3o) according as the carbon carrying the unpaired electron is primary, secondary and tertiary.
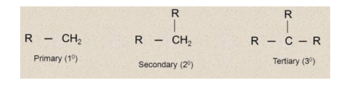
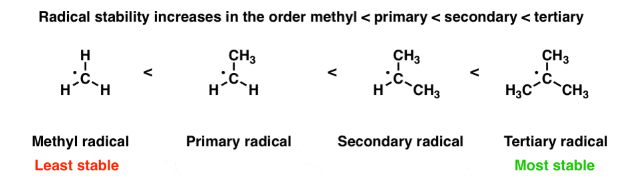
Stability of free radicals
The order of stability of free radicles is the same as that of carbocations i.e. 3o>2o>1o, this order of stability can be explained on the basis of hyperconjugation.
Greater the number of alkyl groups attached to the carbon atoms carrying the odd electron, greater is the delocalisation of the odd electrons and hence more stable is the alkyl free radical.
Allyl and benzyl free radicals are stabilized by resonance, greater the number of phenyl groups more stable is the free radical.
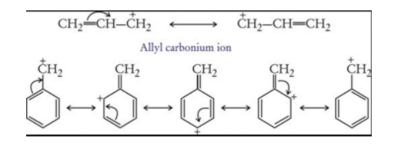
Free radicals are also very short-lived highly reactive chemical species because of the strong tendency of the carbon atom carrying the odd electron to acquire one more electron to complete its octet.
Orbital structure of free Radicles
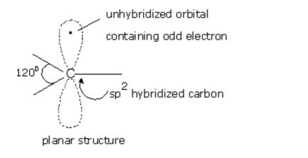
Fig: Structure of Free Radicles
Alkyl free radical are planar chemical species. In free radicals, the unhybridized p-orbital contains the odd electron.
Like carbanions, free radicals can also assume pyramidal shape since the energy difference between planar shape is not much.
Q15) Define Wurtz Reaction?
A15) When an alkyl halide is treated with metallic sodium in the presence of dry diethyl ether, a symmetrical alkane , containing double the number of carbon atoms present in the alkyl group is formed. This reaction is called wurtz reaction.
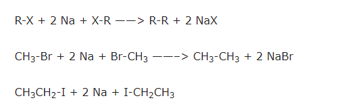
Wurtz reaction is a convenient method for the preparatio of symmetrical alkanes i.e. alkanes containing even number of carbon atoms.
If two different alkyl halides are used to prepare an alkane with odd number of carbon atoms,a mixture of three alkanes is actually produced. This is due to the reason that the twoalkyl halides in addition to reacting with each other also react among themselves giving a mixture of three alkanes.


The boiling point of these alkanes are very close and hence they cannot be separated by fractional distillation. It is because of this drawback that Wurtz reaction is used for the synthesis of only symmetrical alkanes and not for the preparation of unsymmetrical alkenes, i.e., alkanes containing odd number of carbon atoms.
Q16) Define Halogenation in detail?
A16) Mechanism of Halogenation
Halogenation of alkanes occurs by a free radical mechanism.
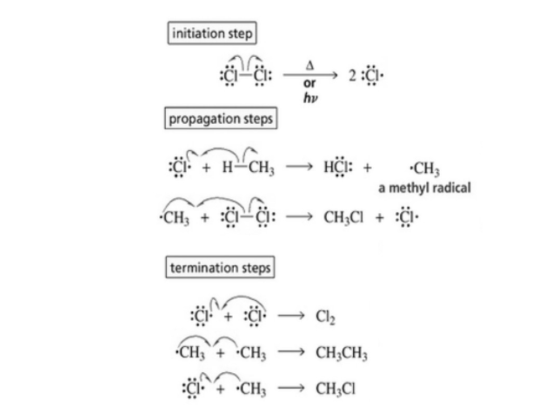
a. Chain initiation: when a mixture of CH4 and Cl2 is heated to 520-670 K in dark or is subjected to UV at room temperature. Cl2 absorbs energy and undergoes homolytic fission producing chlorine free radicals.
b. Chain propagation: each propagation step consists of two reactions.
In the first reaction, the. Cl attacks the CH4 molecule and abstracts a hydrogen atom forming .CH3 and a molecule of HCl.
In the second reaction .CH3 thus produced reacts further with a molecule of Cl2 forming a molecule of methyl chloride and another.Cl. The newly formed. Cl reacts with another
Molecule of CH4 to produce another molecule of HCL and another .CH3. This .CH3 can again repeat reaction. A single photon of light absorbed by Cl2 can bring about the conversion of a large number of CH4 molecules into CH3Cl. Such reactions are called chain reactions.
When sufficient amount of methyl chloride has been formed, the .Cl produced in the reaction has a greater chance of colliding with a molecule of CH3Cl rather than a molecule of CH4, if such a collision occurs, a new free radicle is produced which may subsequently react with Cl2 producing molecule of CH2Cl and another .Cl. This process continuous till all the hydrogen atoms of methane are replaced by halogen atoms.
C . Chain termination : The chain reaction dicussed above may come to a halt if two of the same or different free radicals combine amongst themselevs without producing few free radicals.
Q17) Define a Wurtz-Fittig Reaction?
A17) The Wurtz-Fittig reaction mechanism can be explained either via the organo-alkali mechanism or the radical mechanism. The chemical reaction of aryl halides with alkyl halides and sodium metal with dry ether present to yield substituted aromatic compounds is called the Wurtz-Fittig reaction for the scientists – Charles Adolphe Wurtz and Wilhelm Rudolph Fittig (Wurtz for the discovery of the carbon-carbon bond formation from the coupling of two alkyl halides and Fittig for extending the approach to alkyl halide – aryl halide coupling). An example of such a reaction is shown below.
Wurtz-Fittig Reaction Mechanism
The Organo Alkali Mechanism
When the aryl halide is reacted with sodium metal, an intermediate organo-alkali compound is formed, which is followed by a nucleophilic attack of the alkyl halide as shown below. Thus, the required alkyl-aryl is formed.
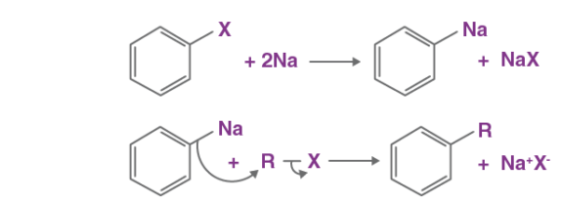
The Radical Mechanism
The sodium atom acts as a moderator for the formation of alkyl radicals and aryl radicals. These alkyl and aryl radicals now combine to form a substituted aromatic compound as shown below.
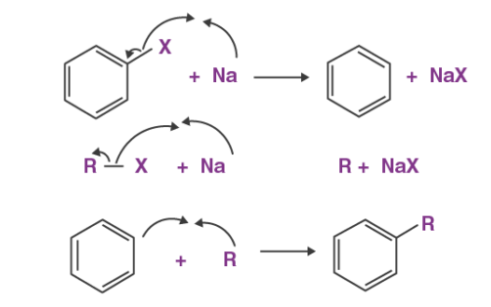
Q18) Define an Elimination reaction?
A18) An elimination reaction is one that involves the loss of two atoms or group of atoms from the same or adjacent atoms of a substance leading to the formation of a multiple bond. Depending on the relative positions of the atoms or groups eliminated, these reactions are classified as alpha α, beta β, gamma ϒ elimination reaction.
1.α -Elimination reaction
The loss of two atoms or groups occur from the same atom of the substrate molecule. For Ex: Base-catalysed dehydrogenation of chloroform to form dichlorocarbene.
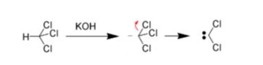
2. β- Elimination reaction
The loss of two or more atoms or groups occur from the adjacent atoms of the substrate molecule. For Ex Acid - catalyst dehydration of alcohols and base-catalyst of dehydrohalogenation of Alkyl halides.
Base catalysed dehydrohalogenation of alkyl halides
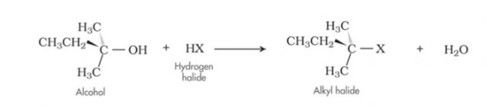
3.ϒ – Elimination
In these reactions, loss of two atoms or group of atoms occurs from α and ϒ position of the molecules leading to the formation of three membered rings.
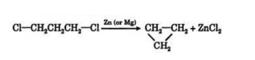
Q19) Define Substitution Reactions?
A19) A substitution reaction is that which involves the direct replacement of an atom or a group of atoms in an organic molecule by another atom or group of atoms without change in the remaining part of the molecule.
The product obtained as a result of substitution is called substitution product and the new atom or group of atoms which enters the molecule is called a substituent.
Depending on the nature of the attacking species the substitution reaction are of the following three types
1.Nucleophilic Substitution Reactions
Substitutions that are brought about by nucleophiles are called nucleophilic substitution reactions. A stronger nucleophile usually displaces a weaker one.
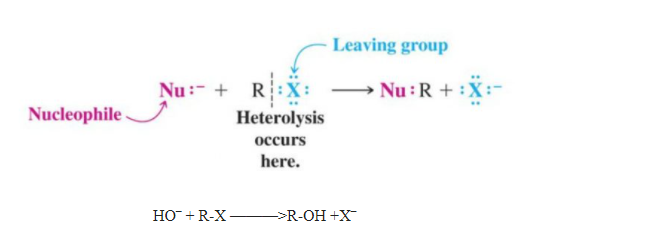
Q20) Define a Carbanion?
A20) A carbanion can be defined as a negatively charged ion in which a carbon atom exhibits trivalence (implying it forms a total of three bonds) and holds a formal negative charge whose magnitude is at least -1. When pi delocalization does not occur in the organic molecule (as it does in the case of aromatic compounds), carbanions typically assume a bent, linear, or a trigonal pyramidal molecular geometry. It is important to note that all carbanions are conjugate bases of some carbon acids.
In all carbanions, the electron density is highly concentrated at the negatively charged carbon atom. Therefore, this carbon becomes an ideal point of attack for many electrophiles and other electron-deficient species. Furthermore, this carbon atom is also the site at which the molecule reacts with proton donors and halogenating reagents such as diiodine.
An illustration detailing the possible resonance structures of a carbanion in which the carbon holding the negative charge is bound to three different R groups is provided above. It can be noted that each of the R-groups in this illustration can either denote an alkyl group, an aryl group, or a hydrogen atom.
Occurrence and Properties
Typically, carbanions behave as nucleophiles and are basic in nature (their pH is usually above 7). The nucleophilicity and the basicity of the carbanion is usually determined by the substituent groups that are attached to the negatively charged carbon. As is the case with most charged carbon species, the substituent groups can either increase or decrease the stability of the molecule as a whole. The effects that come into play while considering the stability of carbanions based on the substituent groups attached to them include:
- The inductive effect, via which highly electronegative substituent groups attached to the carbanion help subdue the negative charge on it and make the molecule more stable. On the other hand, highly electropositive substituent groups can increase the negative charge on the carbanion and, therefore, decrease the overall stability of the molecule.
- The resonance effect, via which the delocalization of the electrons distributes the negative charge all over the carbanion, adding stability in the process. Aromatic systems add a great deal of stability to carbanions when they are present as a substituent group as a result of the resonance effect (and the greater extent of delocalization of electrons over the aromatic system)
- The conjugation of the carbanion.