Unit - 3
Transition Elements-II, Lanthanoids and Actinoids
Q1) What are D Block Elements?
A1)
The elements in the D block are those that can be found in the current periodic table from the third to the twelfth groups. These elements' valence electrons are located in the d orbital. Transition elements or transition metals are other names for D block elements. The following article contains the first three rows of the d block elements, which correspond to the 3d, 4d, and 5d orbitals, respectively.
D block elements are those that have electrons (1 to 10) in the d-orbital of the penultimate energy stage and in the outermost ‘s' orbital (1-2). Despite the fact that electrons do not occupy the ‘d' orbital in group 12 metals, their chemistry is close to that of the preceding groups in several respects, and they are thus classified as d block elements.
Metallic properties such as malleability and ductility, high electrical and thermal conductivity, and strong tensile strength are characteristic of these elements. The d block has four sequence that correspond to the filling of 3d, 4d, 5d, or 6d orbitals.
- 3d- Sc, Ti, V, Cr, Mn, Fe, Co, Ni, Cu, Zn
- 4d- Y, Zr, Nb, Mo, Tc, Ru, Rh, Pd, Ag, Cd
- 5d- La, Hf, Ta, W, Re, Os, Ir, Pt, Au, Hg
- 6d- incomplete.
There are 10 elements filling up the ‘d’ orbital in each series.
Q2) Explain the Position of D Block Elements in Periodic Table?
A2)
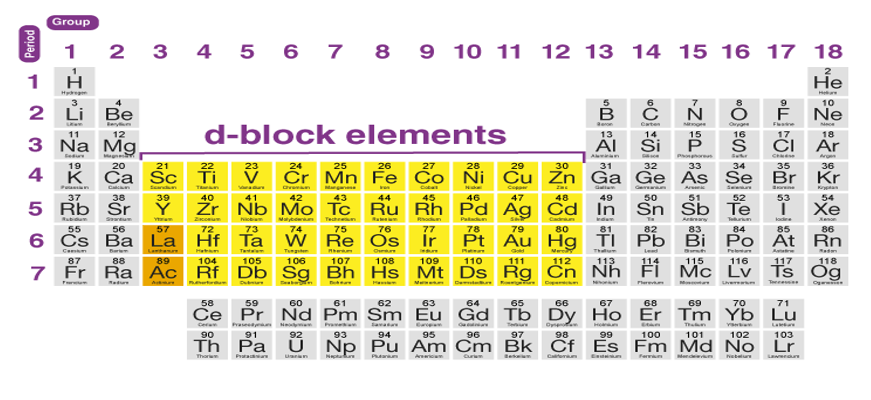
Columns 3 to 12 are occupied by D block elements, which can have atoms with fully filled ‘d' orbitals. A transition metal is described by the International Union of Pure and Applied Chemistry as "an element whose atom or cations has a partially filled d sub-shell."
Q3) Why D Block Elements are called Transition Elements? Explain the Properties of Transition Metals.
A3)
Groups 4–11 are made up of transition elements. Transition elements include scandium and yttrium from Group 3, which have a partially filled d subshell in the metallic state. Elements in the 12 columns of the d block, such as Zn, CD, and Hg, have fully filled d-orbitals and are thus not called transition elements.
Transition Elements get their name from the fact that they are placed between s and p block elements and have properties that transition between them. So, although all transition metals are d block elements, they are not all transition elements.
Properties of Transition Metals
1. Electrons are attached to the ‘d' sub-orbitals, which are located between the (n+1) s and (n+1) p sub-orbitals.
2. In the periodic table, it is located between the s and p block elements.
3. The differences in properties between the s and p-block elements.
Q4) Explain Electronic Configuration of D Block Elements.
A4)
The electronic configuration of D block elements is (n-1) d 1-10ns 1-2. Half-filled orbitals and fully filled d orbitals are both stable for these elements. The electronic structure of chromium, which has half-filled d and s orbitals in its configuration – 3d54s1, is an example of this. Another example is the electronic configuration of copper. Copper has a 3d104s1 electronic structure rather than a 3d94s2.
The relative stability of the fully filled d orbital can be due to this. In both their ground and general oxidation states, zinc, mercury, cadmium, and copernicium have fully filled orbitals. As a result, these metals aren't classified as transition elements, whereas the others are classified as d block elements.
1. For time 4, transition components, the electronic configuration is (Ar) 4s 1-2 3d 1-10.
2. For time 5, transition elements, the electronic configuration is (Kr) 5s 1-2 4d 1-10.
3. For time 6, transformation components, the electronic configuration is (Xe) 4s 1-2 3d 1-10.
According to the Aufbau principle and Hund's rule of multiplicity, electrons are added to the 3d subshell from left to right along the time.
1st transition series | Sc | Ti | V | Cr | Mn | Fe | Co | Ni | Cu | Zn |
4s23d1 | 4s23d2 | 4s23d3 | 4s13d5 | 4s23d5 | 4s23d6 | 4s23d7 | 4s23d8 | 4s13d10 | 4s23d10 | |
2nd transition series | Y | Zr | Nb | Mo | Tc | Ru | Rh | Pd | Ag | Cd |
5s24d1 | 5s24d2 | 5s14d4 | 5s14d5 | 5s24d5 | 5s14d7 | 5s14d8 | 5s04d10 | 5s14d10 | 5s24d10 | |
3rd transition series | La | Hf | Ta | W | Re | Os | Ir | Pt | Au | Hg |
6s25d1 | 6s25d2 | 6s25d3 | 6s25d4 | 6s25d5 | 6s25d6 | 6s25d7 | 6s15d9 | 6s15d10 | 6s25d10 |
Many of the series have anomalies, which can be explained by the following factors.
1. The difference in energy between the ns and (n-1) d orbitals.
2. Pairing energy for s-orbital electrons
3. Half-filled orbitals are stable as compared to partially filled orbitals.
Chromium has a 4s13d5 electron configuration rather than a 4s23d4 electron configuration, while copper has a 4s13d10 electron configuration rather than a 4s23d9. The stability of half-filled orbitals compared to partially filled orbitals explains these inconsistencies in the first transition sequence.
From niobium onwards, electron presence in d orbitals tends to be favoured over electron sharing in s orbitals in the second sequence of transition metals. The electron can choose between sharing in the s orbital or being excited to the d orbital from the available s and d orbitals. Obviously, the option is determined by the amount of repulsive energy overcome during sharing and the energy difference between the s and d-orbitals.
Since the s and d-orbitals have almost the same energy in the second series, electrons tend to occupy the d-orbital. As a result, s-orbital has only one electron in niobium. Transition metals in the third sequence, on the other hand, have a higher number of paired s configurations, even at the cost of half-filled orbitals (Tungsten- 6s25d4). This sequence follows the filling of 4f orbitals and the lanthanide contraction that follows.
Because of the smaller scale, the ‘f' electron provides a lot of shielding for the d orbitals. The energy difference between the s and 5d orbitals is increased as a result of the shielding, and the pairing energy is less than the excitation energy. Despite the stability provided by half-filled orbitals, tungsten does not allow for electron excitation.
Q5) Explain Atomic and Ionic Radii of D Block Elements.
A5)
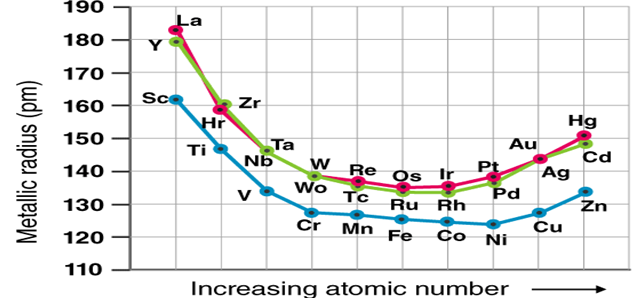
Metallic Radii of 1st, 2nd, and 3rd Row Transition Metals
Atomic and ionic radii of elements of all three-transition series
1. Rapidly decreases from column 3 to column 6.
2. From column 7 to 10, it stays the same, and 3. From column 11 to 12, it starts to increase.
For e.g., in the first transition sequence, atomic radii, the decrease is greater from Sc to Cr (group 3 to 6), and is nearly the same for Mn, Fe, Co, Ni (group 7,8, 9 &10), with an increase in Cu and Zn.
1. The greater reduction in atomic radii in column 3 to 6 elements is due to an increase in effective nuclear charge, but insufficient shielding due to the fewer d-electrons.
2. The repulsion between the mutual d electrons in elements in column 7 to 10 balances the increasing effective nuclear charge, resulting in the same radii.
3. The d orbital is filled with ten electrons in 11 and 12 column components, shielding the electrons in the higher s-orbital. As a result, group 11 and 12 elements such as Cu and Zn are larger than the block's earlier elements.
The radii of the third series elements are to be greater than the radii of the second series elements since electrons occupy a higher orbital. However, the radii of both series are nearly identical. The 5d orbitals in the third series elements are filled only after the 4f orbitals have been filled, raising the effective nuclear charge by 14 units.
Lanthanide contraction is a term used to describe the greater shrinkage of radii caused by a higher nuclear charge. The increased nuclear effective charge effectively neutralises the rise in radii due to the higher orbital. As a result, the atomic radii of the second and third series elements are the same. Niobium and hafnium, for example, have almost identical atomic radii.
Q6) Explain the Properties of D Block Elements.
A6)
- Ionization Energy of D Block Elements
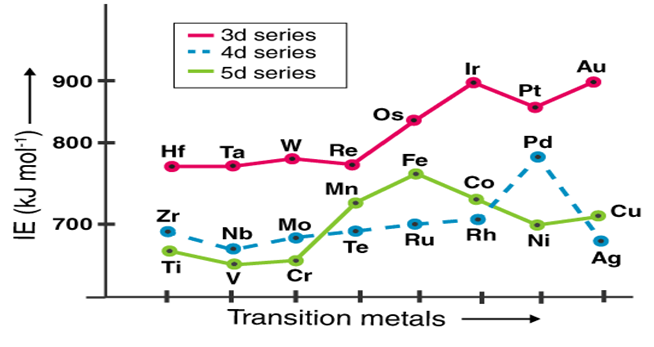
Ionization energy is the amount of energy required to detach the valence electron from an atom/ion, and it is proportional to the electron's force of attraction. As a result, the ionisation energy increases as the nuclear charge and electron radii decrease (IE). For half-filled and completely filled orbitals, the ionisation energy would be higher.
The ionisation energy of the d block elements is higher than that of the s-block and lower than that of the p-block elements, which they are sandwiched between. Except for chromium and copper, the first Ionization Energy sequence requires removal from a filled s-orbital. The Ionization Energy of d block elements increases as the atomic number increases up to Fe.
2. Metallic Character
High tensile strength, malleability, ductility, electrical and thermal conductivity, metallic lustre, and crystallisation in bcc/ccp/hcp structures are all characteristics of D block elements.
Except for Copper, they are extremely hard and have a high enthalpy of atomization and low volatility. The number of unpaired electrons increases the hardness. As a result, among the d block elements, Cr, Mo, and W are extremely hard metals. In this regard, the group-12 elements (Zn, Cd, and Hg) are also an exception.
3. Oxidation States of D Block Elements
The oxidation state is a hypothetical state in which the atom tends to lose or gain more electrons than it does in its normal valency state. It's also useful for demonstrating the atom's/properties. Ion's Both s and d-orbitals may have electrons in transition elements/ions.
Since the energy difference between the s and d orbitals is small, both electrons will participate in the formation of ionic and covalent bonds, resulting in multiple(variable) valency states (oxidation states).
4. Trends in the Oxidation States
Cr, Cu, Ag, Au, and Hg all have a minimum Oxidation state of 1.
2. Oxidation state becomes more stable in the order 3d 4d 5d. The elements of the 3d series are the most stable in +2, the 4d series in +2 and +4, and the 5d series in +4. In their higher OS, Cr6+ and Mn7+ (of 3d) are not stable. CrO42- and MnO4– containing compounds are highly reactive and strong oxidising agents.
In their higher OS, Mo6+ and Tc7+ (of 4d) are stable. MoO42- and TcO4– containing compounds are unreactive and stable. W6+ and Re7+ (of 5d) are similarly stable in their higher OS. Compounds containing them, such as WO42- and ReO4–, are safe and unreactive.
Q7) Explain Physical Properties of D Block Elements
A7)
Density: Among the transition series, the trend in density will be reverse of atomic radii, i.e., density increase remains almost the same and then decreases along the period.
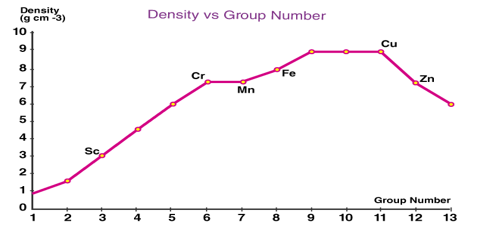
When you go down the column, the column density of the 4d series is higher than that of the 3d series. Due to lanthanide contraction and a greater decrease in atomic radii, the volume density of transition elements in the 5d series is double that of the 4d series.
In the 3d series, scandium has the lowest density, while copper has the highest density. Osmium (d=22.57g cm-3) and Iridium (d=22.61g cm-3) are the 5d sequence elements with the highest density of all the d block elements.
Some d block elements with relative radii are Fe Ni Cu, Fe Cu Au, and Fe Hg Au.
Q8) Why D Block Elements have high Melting and Boiling Point?
A8)
In addition to the metallic bonding formed by s-electrons, unpaired electrons and empty or partially filled d-orbitals form covalent bonding. D-block elements have higher melting and boiling points than s and p block elements due to their heavy bonding. This pattern continues until the d5 configuration, after which it reverses as more electrons in the d-orbital pair.
1. In their sequence of elements, Cr, Mo, and W have the highest melting at boiling point.
2. The half-filled configuration of manganese (Mn) and technetium (Tc) results in poor metallic bonding and abnormally low melting and boiling points.
3. There are no unpaired d-electrons in Group 12, Zn, Cd, or Hg, because there is no covalent bonding. They will have the lowest melting and boiling points in the game.
Mercury is the only metal that can remain in a liquid state at room temperature. Mercury's 6s valence electrons are more closely pulled by the nucleus (lanthanide contraction), resulting in less involvement of outer s-electrons in metallic bonding.
Q9) What Transition Elements are Considered Noble Metals?
A9)
In the three-transition series
1. The ionisation energies of elements gradually increase over a row.
2. From the left corner of the 3d series to the right corner of the 5d transition elements, density, electronegativity, electrical, and thermal conductivities increase, while metal cation hydration enthalpies decrease.
This means that the transition metals are gradually becoming less reactive and more "noble" in nature. Metals (Pt, Au) in the lower right corner of the d block have such high ionisation energies, increasing electronegativity, and decreasing low enthalpies of hydration that they are often referred to as "noble metals."
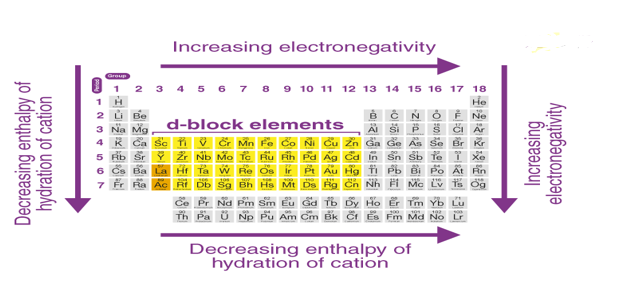
Q10) Write short note on Magnetic Properties of D Block Elements
A10)
The interaction of materials with the magnetic field is defined as follows:
If it is repelled, it is diamagnetic; if it is attracted, it is paramagnetic; and if it is ferromagnetic, it will maintain its greater magnetic nature even in the absence of a magnetic field.
Diamagnetism is caused by paired electrons. Para-magnetism is caused by unpaired electrons, whereas ferromagnetism is caused by unpaired electrons aligned together. Depending on the unpaired electrons, D block elements and their ions show this behaviour.
The ‘orbital magnetic moment' and the ‘spin magnetic moment' are both influenced by unpaired electrons. The orbital angular moment is negligible in the 3d series, so the estimated spin-only magnetic moment is given by the formula:
= [4s (s + 1)] = [4s (s + 1)] = [4s (s + 1)] = [4s (s [n (n + 1)] = [n (n + 1)] = [n (n + 1)] = [n (BM
Where ‘n' is the number of unpaired electrons and ‘S' is the total spin. Bohr Magneton is its unit (BM). The real magnetic moment for higher d-series involves elements from the orbital moment as well as the spin moment. The maximum number of unpaired electrons and magnetic moment are found in chromium and molybdenum.
Ion | Outer configuration | No. Of unpaired electrons | Magnetic moment (BM) | |
Calculated | Observed |
|
|
|
Sc3+ | 3d0 | 0 | 0 | 0 |
Ti3+ | 3d1 | 1 | 1.73 | 1.75 |
Ti2+ | 3d2 | 2 | 2.84 | 2.86 |
V2+ | 3d3 | 3 | 3.87 | 3.86 |
Cr2+ | 3d4 | 4 | 4.90 | 4.80 |
Mn2+ | 3d5 | 5 | 5.92 | 5.95 |
Fe2+ | 3d6 | 4 | 4.90 | 5.0-5.5 |
Co2+ | 3d7 | 3 | 3.87 | 4.4-5.2 |
Ni2+ | 3d8 | 2 | 2.84 | 2.9-3.4 |
Cu2+ | 3d9 | 1 | 1.73 | 1.4-2.2 |
Zn2+ | 3d10 | 0 | 0 | 0 |
Q11) Explain the Formation of Coloured Ions by D Block Elements
A11)
D block element compounds come in a number of colours. When a frequency of light is absorbed, the light emitted has a complementary colour to the absorbed frequency. Transition element ions can absorb visible frequency and use it in two ways, resulting in visible colour.
d-d Transition
Excitation of an electron to a higher energy level is one process. The presence of a d-electron and an empty d-orbital in transition element ions can result in colour formation. Excitation and de-excitation of valence electrons. This is referred to as a d-d transition.
D-orbitals are degenerate and have the same energy as D-orbitals. The presence of ligands capable of forming coordinate bonds with these ions removes the degeneracy and divides the d-orbitals into two groups: e.g., and t2g. The intensity of the incoming ligand determines the energy difference (E).
By absorbing energy in the visible region (=400-700nm) and transmitting (giving) a complementary colour, electrons in the lower d-orbitals can be excited into the higher d-orbitals.
[Cu (H2O)6] 2+ ions, for example, absorb red radiation and look complementary blue-green. In the sunlight, hydrated Co2+ ions absorb radiation in the blue-green zone and look red.
Cupric ion is colourless and in the presence of water molecules becomes blue in colour.
a) The colour of the ion’s changes depending on their oxidation state. Cr6+ is yellow in colour, similar to potassium dichromate, while Cr3+ and Cr2+ are green and blue, respectively.
b) The compound's colour is also affected by the complexing or coordinating group. Cu2+, for example, has a light blue colour when it is liganded with water, but a deep blue colour when it is liganded with ammonia.
c) Transition metal ions, which have the following properties:
1. D-orbitals that are fully filled and have no empty d-orbitals for electron excitation are colourless. Cu+(3d10), Zn2+(3d10), Cd2+(4d10), and Hg2+(5d10) are colourless metals.
2. Colourless transition metal ions are those with fully empty d-orbitals and no d-electrons. The ions Sc3++(3d0) and Ti4++(3d0) are colourless.
d – p bonding L-M and M-L
Ligands will donate their p electrons to metal ions' empty d orbitals. This interaction, also known as ligand-metal / metal-ligand or d – p bonding, can give compounds colour.
Q12) What is the Important Compounds of D Block Elements.
A12)
The D Block Elements are used to make a variety of important industrial compounds. Some examples of such compounds are:
1. K2Cr2O7 (Potassium Dichromate): In the leather industry, this compound is extremely essential. In most azo compound preparation methods, it is often used as an oxidant.
The dichromate ion is made up of two tetrahedra that share a single corner with a bond angle of 1260 between Chromium, Oxygen, and Chromium. Potassium dichromate is a strong oxidizer. In the method of volumetric analysis, potassium dichromate is also used as a primary norm.
KMnO4 is the second element in the KMnO4 formula (Potassium Permanganate)
The physical appearance of KMnO4 is intensely purple. It has diamagnetic properties as well as poor paramagnetic properties that are temperature dependent.
The lack of unpaired electrons in the permanganate ion causes diamagnetism. In organic chemistry, potassium permanganate is often used as an oxidant in the preparation of various materials. It can also be used to bleach cotton, silk, and wool. Because of its high oxidising capacity, it can also be used to decolorize oils.
Neutral-Atom Electron Configurations
It's easy to figure out which electrons are in which orbitals by counting through the periodic table. As previously mentioned, the number of electrons in a neutral atom can be determined by counting protons (atomic number). This process can be sped up by organising by block. Please refer to the section on electron configuration if you have any doubts about this counting system or how electron orbitals are filled.
For instance, if we wanted to figure out the electronic structure of Vanadium (atomic number 23), we'd start with hydrogen and work our way down the Periodic Table.
1s (H, He), 2s (Li, Be), 2p (B, C, N, O, F, Ne), 3s (Na, Mg), 3p (Al, Si, P, S, Cl, Ar), 4s (K, Ca), 3d (H, He, Li, Be, Li, Be, Li, Be, Li, Be, Li, Be, Li, Be, Li, Be, Li, Be, Li, Be, Li, Be, Li, Be (Sc, Ti, V).
This organisation is verified by looking at the periodic table below. In the third orbital, we have three components. As a result, we write the orbitals in the order in which they were filled.
1s2 2s2 2p6 3s2 3p6 4s2 3d3 1s2 2s2 2p6 3s2 3p6 4s2 3d3
Or
4s2 3d3 [Ar]
The neutral atom configurations of the fourth period transition metals are in Table 22.
Ti | V | Cr | Mn | Fe | Co |
[Ar] 4s23d2 | [Ar] 4s23d3 | [Ar] 4s23d4 | [Ar] 4s23d5 | [Ar] 4s23d6 | [Ar] 4s23d7 |
|
| [Ar] 4s13d5 |
|
|
|
Copper and chromium tend to be outliers. Take a quick glance at the Periodic Table to see where the element Chromium (atomic number 24) falls (Figure 11). Chromium has the electronic structure [Ar] 4s13d5 rather than [Ar] 4s23d4. Since a partially filled 3d manifold (with one 4s electron) is more stable than a partially filled d-manifold, this is the case (and a filled 4s manifold). Table 22 shows that the copper experiences a similar anomaly, despite having a totally filled d-manifold.
Multiple Oxidation States
Since transition metals are relatively quick to lose electrons compared to alkali metals and alkaline earth metals, most of them have several oxidation states. The valence s-orbital of alkali metals has one electron, and their ions almost always have oxidation states of +1. (From losing a single electron). Alkaline earth metals, on the other hand, have two electrons in their valence s-orbitals, resulting in ions with a +2-oxidation state (from losing both). Transition metals, on the other hand, are more complex, with a wide variety of detectable oxidation states due to the removal of d-orbital electrons. The most common oxidation states of period 3 elements are depicted in the chart below.
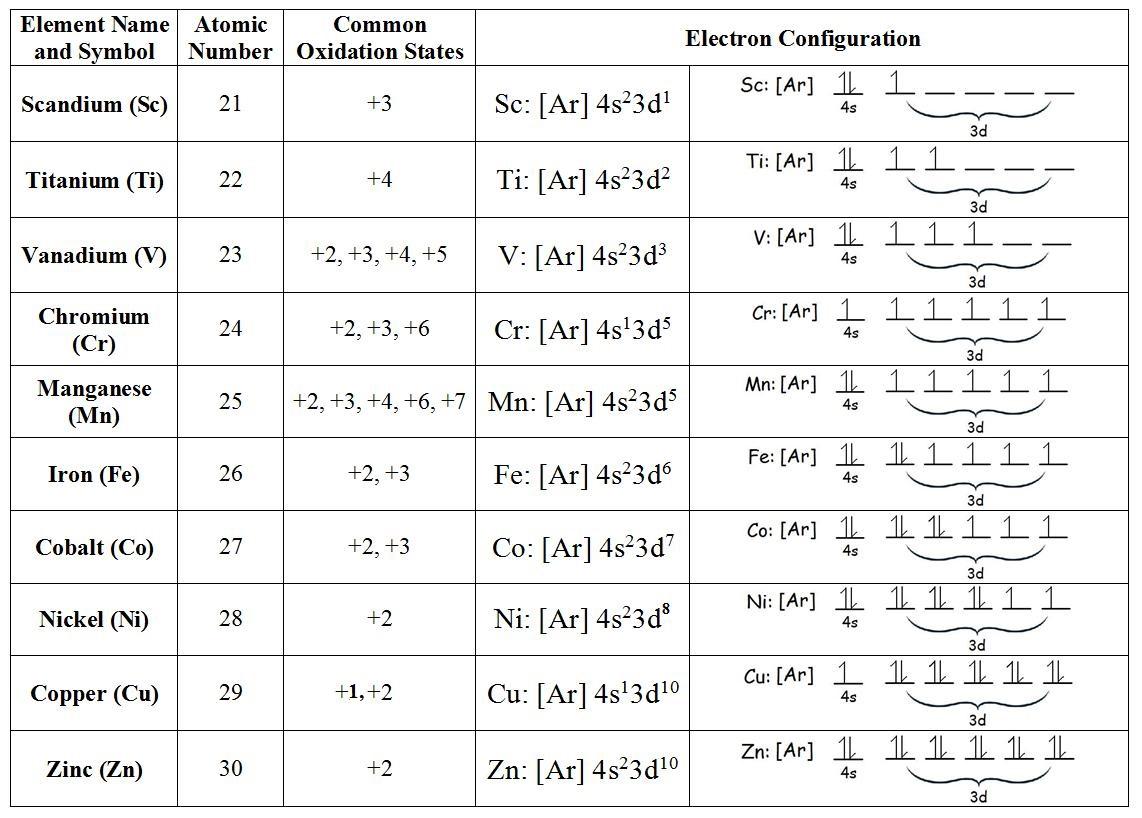
Scandium is one of only two transition metal elements with only one oxidation state (the other being zinc, which has an oxidation state of +2). The oxidation states of all the other elements are at least two. Manganese, which is found in the middle of the era, has the most oxidation states, and therefore the highest oxidation state in the entire period, since it contains five unpaired electrons (see table below).
It's important to note the pattern when it comes to the stability of higher oxidation states for transition metals: the stability of higher oxidation states gradually increases down a group. For example, Cr is most stable at a +3-oxidation state in group 6, which means that stable Cr forms in the +4 and +5 oxidation states are rare. At +4 and +5 oxidation states, however, there are several stable forms of molybdenum (Mo) and tungsten (W).
Q13) What are F Block Elements?
A13)
The elements of the F block are divided into two groups: lanthanoids and actinoids. These elements are known as inner transition metals because they provide a transition between the s and d blocks of the periodic table in the 6th and 7th rows.
F block elements are those that have their f orbital packed with electrons. These elements have electrons in the f orbital (1 to 14), the penultimate energy level's d orbital (0 to 1), and the outermost orbital.
The f block has two sequence that led to the filling of 4f and 5f orbitals. The components are the Ce to Lu 4f series and the Th to Lw 5f series. In each sequence, the ‘f' orbital is filled with 14 elements.
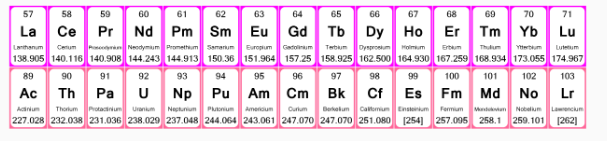
The position of F Block Elements in the Periodic Table: F block elements are
Put at the bottom of the periodic table separately They're a combination of the 6th and 7th periods.
Q14) Explain the Classification of F Block Elements.
A14)
The elements of the f block are further classified as follows:
1. The first group of elements is known as the lanthanides, and it consists of elements with atomic numbers ranging from 57 to 71. These are non-radioactive elements (except for promethium, which is radioactive).
2. Actinides are the second group of elements, which includes elements with atomic numbers ranging from 89 to 103. The majority of these elements are radioactive.
The following is a list of all the f block elements. The row that starts with Lanthanum contains all of the lanthanides, while the row that starts with Actinium contains all of the actinides.
Q15) Explain the Properties of F block Elements.
A15)
1. Add electrons to the (n-2) level 2's 'f' sub-orbitals. In the periodic table, they are found between the (n-1) d and ns block elements.
2. Properties are comparable to those of d-block elements.
Lanthanides vs. Actinides: What's the Difference?
1. The filling of 4f-orbitals is done by lanthanoids, whereas the filling of 5f-orbitals is done by actinoids. The binding energy of 4f electrons is lower than the binding energy of 5f electrons. When compared to 4f-electrons, the shielding effect of 5f-electrons is less efficient.
2. The paramagnetic properties of lanthanoids are simple to describe, but actinoids are more difficult to explain.
3. Lanthanides, with the exception of promethium, are non-radioactive in nature, whereas all actinide sequence elements are radioactive.
4. While lanthanides have a low proclivity for forming oxo-cations, the actinide sequence has many oxo-cations. The compounds formed by lanthanides are less basic than those formed by actinides, which are extremely basic.
Q16) What are Lanthanides?
A16)
Lanthanides are the rare earth elements in the current periodic table, which include elements with atomic numbers ranging from 58 to 71 after Lanthanum. Rare earth metals are so called because they only make up a small percentage of the Earth's crust (310-4 percent). As lanthanide orthophosphates, they can be found in ‘monazite' sand. In the year 1925, the Norwegian mineralogist Victor Goldschmidt coined the word "lanthanide." All but one of the fifteen metallic elements in the lanthanide family (from lanthanum to lutetium) are f-block elements. These elements' valence electrons are in the 4f orbital. Lanthanum, on the other hand, is a d-block element with a [Xe]5d16s2 electronic configuration.
Lanthanides are extremely dense elements, ranging in density from 6.1 to 9.8 grammes per cubic centimetre. These elements, like most metals, have extremely high melting and boiling points (ranging from 800 to 1600 degrees Celsius) (ranging from roughly 1200 to 3500 degrees Celsius). Ln3+ cations are known to form in all lanthanides.
Lanthanides are extremely dense metals with melting points that are much higher than those of the d-block elements. They combine with other metals to form alloys. These are the inner transition metals, which are also known as the f block elements. In the inner transition elements/ions, electrons can be found in the s, d, and f orbitals.
Q17) Explain the Properties of Lanthanide Series
A17)
If we consider the lanthanides and actinides series in the periodic table for transition metals, the table would be too broad. These two series, known as the 4f series (Lanthanoids series) and 5f series, are found at the bottom of the periodic table (Actinoids series). Inner transition elements are the 4f and 5f series put together.
In terms of chemical and physical properties, all of the elements in the sequence are very similar to lanthanum. The following are some of the most important characteristics and properties:
1. They have a lustrous sheen to them and look silvery.
2. They're made of soft metals that can be sliced with a knife.
3. Depending on their basicity, the elements have different reaction tendencies. Some people are quick to respond, while others take their time.
4. If contaminated with other metals or non-metals, lanthanides will corrode or become brittle.
5. They almost all combine to form a trivalent compound. They can also form divalent or tetravalent compounds on occasion.
6. They are attracted to each other.
Q18) What Consequences of Lanthanide Contraction
A18)
The effect of lanthanide contraction will be clearly depicted in the following points:
1. Atomic mass
2. The separation of lanthanides is difficult.
3. Hydroxide
4. The formation is complicated.
5. The d-block elements' ionisation energy
1. Atomic size: The third transition series atom is almost identical in size to the second transition series atom. For e.g., the radius of Zr equals the radius of Hf, and the radius of Nb equals the radius of Ta, and so on.
2. Difficulty in the separation of lanthanides: Lanthanides' chemical properties are identical since their ionic radii differ just slightly. This makes it difficult to separate elements in their pure state.
3. Effect on the basic strength of hydroxides: The covalent character of the hydroxides increases as the size of lanthanides decreases from La to Lu, and thus their basic strength decreases. As a result, La (OH)3 is the most basic, while Lu (OH)3 is the least basic.
4. Complex formation: The propensity to shape coordinates is due to the smaller size but higher nuclear charge. From La3+ to Lu3+, the number of complexes increases.
5. Electronegativity: From La to Lu, it gets better.
6. Ionization energy: The nuclear charge attracts electrons much more strongly, so the ionisation energy of 5d elements is much higher than that of 4d and 3d elements. Except for Pt and Au, all elements in the 5d sequence have a filled s-shell.
Ionization Energy is the same for all elements from Hafnium to Rhenium, and it increases with the amount of mutual d-electrons after that, with Iridium and Gold having the highest Ionization Energy.
Case Study:
Mercury – the liquid metal: At room temperature, mercury is the only metal that remains in its liquid form. Mercury's 6s valence electrons are more closely pulled by the nucleus (lanthanide contraction), resulting in less involvement of outer s-electrons in metallic bonding.
7. Formation of Complex: Lanthanides with a 3+ oxidation state has a higher charge to radius ratio and hence a lower charge to radius ratio. As compared to d-block elements, this decreases the ability of lanthanides to form complexes. They still form complexes with strong chelating agents such as EDTA, -diketones, and oxime, among others. P-complexes are not formed by them.
Q19) Explain Oxidation State of Lanthanides
A19)
The oxidation state of all elements in the lanthanide sequence is +3. Some metals (samarium, europium, and ytterbium) were previously thought to have +2 oxidation states. Further research on these metals and their compounds has shown that in solution, all metals in the lanthanide sequence have a +2 oxidation state.
A few metals in the lanthanide sequence have +4 oxidation states on rare occasions. The high stability of empty, half-filled, or completely filled f-subshells is responsible for the uneven distribution of oxidation states among metals.
The oxidation state of lanthanides is affected by the stability of the f-subshell in such a way that the +4 oxidation state of cerium is preferred because it acquires a noble gas configuration, but it reverts to a +3 oxidation state and thus acts as a strong oxidant that can also oxidise water, though the reaction is slow.
The oxides of: display the +4 oxidation state as well.
Praseodymium is the first element in the periodic table (Pr)
2. The element neodymium (Nd)
Terbium is the third element of the periodic table (Tb)
Dysprosium is a kind of dysprosium (Dy)
Europium (atomic number 63) has the electronic configuration [Xe] 4f7 6s2, which means it loses two electrons from the 6s energy level and achieves the extremely stable, half-filled 4f7 configuration, which makes it easy to shape Eu2+ions. Eu2+ then oxidises to the normal lanthanide oxidation state (+3) and forms Eu3+, which acts as a strong reducing agent.
Ytterbium In the Yb2+ state, (atomic number 70) has a totally filled f-orbital and is a good reducing agent for similar reasons.
The existence of an f-subshell has a significant impact on the oxidation state and properties of these metals. New discoveries and advances continue to contribute to the body of knowledge about lanthanides.
Unlike the d-block elements, the energy gap between 4f and 5d orbitals is high, limiting the number of oxidation states.
Q20) Explain Physical Properties of Lanthanides.
A20)
1. Density: Since density is defined as the ratio of a substance's mass to its volume, d-block elements will have a higher density than s-block elements. The density pattern in the inner transition sequence will be the inverse of atomic radii, i.e., density will increase as the atomic number increases over time.
Their density is high, ranging from 6.77 to 9.74 g cm-3. It rises as the number of atoms in the nucleus rises.
2. Melting and Boiling Points: Lanthanides have a relatively high melting point, but there is no clear trend in their melting and boiling points.
3. Magnetic Properties: The interaction of materials with the magnetic field is defined as follows:
1. If repelled, diamagnetic
2. If attracted, paramagnetic
Because of unpaired electrons in orbitals, lanthanide atoms/ions other than f0 and f14 are paramagnetic. As a result, the diamagnetic elements Lu3+, Yb2+, and Ce4+ exist.
The ‘orbital magnetic moment' and the ‘spin magnetic moment' are both influenced by unpaired electrons. The overall magnetic moment is calculated using the orbital angular moment and spin magnetic moment of the electrons.
Μ = √[4S(S+1) +L(L+1)] BM and its unit is Bohr Magneton (BM)
Q21) What are Actinides?
A21)
The word "actinide sequence" comes from actinium, the first element in the series. The symbol An is used to refer to all of the actinide series elements, which have atomic numbers ranging from 89 to 103 in the periodic table.
Both elements in the actinide sequence are radioactive in nature, and radioactive decay releases a significant amount of energy. The most common naturally occurring actinides on Earth are uranium and thorium, while plutonium is synthesised.
Power reactors and nuclear weapons also use these components. Uranium and thorium are currently used in a variety of applications, while americium is used in the ionisation chambers of modern smoke detectors.
Lanthanides and actinides are shown as two separate rows below the main periodic table in the current periodic table.
The general electronic configuration of actinides is [Rn] 5f1-14 6d0-1 7s2. Here [Rn] is the electronic configuration of the nearest noble gas which is Radium.
Q22) Define Lanthanide contraction.
A22)
All 14 elements in the Lanthanide sequence are affected by the Lanthanide Contraction. Cerium(Ce), Praseodymium(Pr), Neodymium(Nd), Promethium(Pm), Samarium(Sm), Europium(Eu), Gadolinium(Gd), Terbium(Tb), Dysprosium(Dy), Holmium(Ho), Erbium(Er), Thulium(Tm), Ytterbium(Yb), and Lutetium(Yb) are members of this sequence (Lu). According to the Lanthanide Contraction, the atomic radius of these elements decreases as the atomic number increases. A periodic table can be used to compare the elements Ce and Nd. The atomic number of Ce is 58, and the atomic number of Nd is 60. Which one's atomic radius would be smaller? Because of its greater atomic number, Nd would.
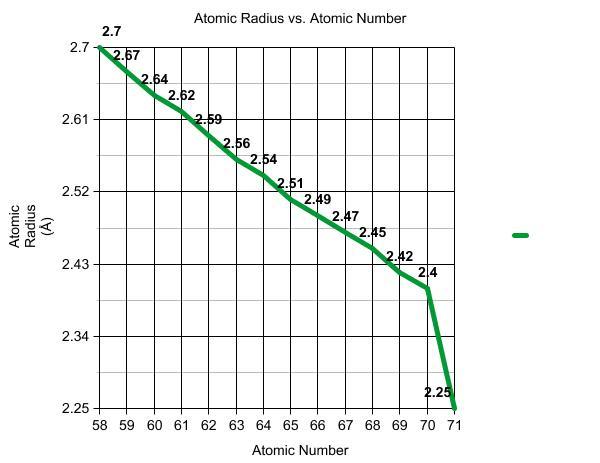
The graph shows the atomic radius decreasing as the atomic number is increasing, Lanthanide Contraction.
● Shielding and its Effects on Atomic Radius
The Lanthanide Contraction is caused by the 4f electrons' weak shielding effect. The shielding effect is a mechanism in which the inner-shell electrons protect the outer-shell electrons from the effects of nuclear charge. If the shielding isn't as strong, the positively charged nucleus attracts the electrons more, reducing the atomic radius as the atomic number rises. The s orbital has the most shielding, the f orbital has the least, and the p and d orbitals are in the centre, with p being greater than d.
When comparing elements with f electrons and those without f electrons in the d block orbital, the Lanthanide Contraction can be observed. Pd and Pt are two examples of such elements. Pd has 4d electrons, while Pt has 5d and 4f. The atomic radius of these two elements is almost identical. Lanthanide Contraction and Shielding are to blame for this. Pt should have a much greater radius when more electrons and protons are added, but it doesn't because the 4f electrons are weak at shielding. Where the shielding is inadequate, there will be a higher nuclear charge, which will draw the electrons in closer together, resulting in a radius that is smaller than predicted.
Row 1 of Periodic Table D block Row 2 Row 3
The graphs show the atomic radii of the transition metals' first three rows. We may apply the same concept to entire rows and columns as we did with the elements Pd and Pt. When we compare Row 1 and Row 2, we can see that the atomic radii of the elements vary significantly, but when we compare Row 2 and Row 3, the atomic radii do not differ significantly. The atomic radii of elements 23 and 41, which are in the same column of the periodic table, differ greatly (atomic radii rise from Row 1 to Row 2), but elements 41 and 73, which are also in the same column, differ only slightly. The introduction of 4f electrons in Row 3 is due to this. We would expect the elements in Row 3 to follow the same pattern as in Rows 1 and 2 (a significant increase in atomic radii), but this is not the case. This is due to the fact that the 4f orbitals aren't very good at shielding.
Q23) Explain General features of actinoids.
A23)
1. Because of their volatility, they are all radioactive.
2. The majority is made synthetically by particle accelerators that produce nuclear reactions and is short-lived.
3. Since they have an atomic number greater than 83, they are both unstable and reactive (nuclear stability).
4. In metallic form, they all have a silvery or silvery-white lustre.
5. They're all capable of forming stable complexes with ligands including chloride, sulphate, carbonate, and acetate.
6. Several actinides can be found in seawater or minerals in nature.
They can undergo nuclear reactions, for example.
8. They are dangerous to treat because of radioactivity emission, toxicity, pyrophoricity, and nuclear criticality.
a. Radioactivity Emission: The elements emit alpha, beta, and gamma radiation, as well as neutrons created by spontaneous fissions or boron, beryllium, and fluorine reacting with alpha-particles.
b. Toxicity: They are considered poisonous elements due to their radioactive and heavy metal properties.
c. Pyrophoricity: Many actinide metals, hydrides, carbides, alloys, and other compounds can spontaneously ignite in a finely divided state at room temperature, resulting in fires and the spread of radioactive contaminates.
d. Nuclear Criticality: When fissionable materials are mixed, a chain reaction may occur, resulting in lethal doses of radioactivity, but this is dependent on chemical structure, isotopic composition, geometry, and the size of the surrounding environment, among other factors.