Unit - 3
Electrochemistry-I
Q1) What is an Electrolytic Cell?
A1) An electrolytic cell is an electrochemical cell that promotes a chemical reaction through the induction of electrical energy.
Electrolysis is the process of carrying out non-spontaneous reactions under the influence of electric energy.
An electrolytic cell is an electrochemical cell that promotes a chemical reaction through the induction of electrical energy.
Electrolysis is the process of carrying out non-spontaneous reactions under the influence of electric energy.
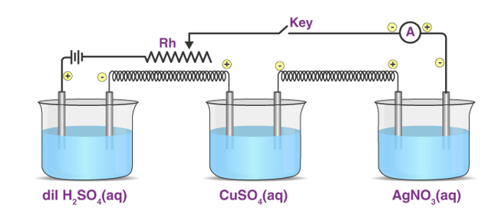
Michael Faraday conducted substantial research on electrolysis of electrolyte solutions and melts. He was the first scientist to describe the Laws of Electrolysis in quantitative terms. He developed two laws to explain the quantitative characteristics of electrolysis, which are today known as Faraday's laws of electrolysis, namely the first and second laws of electrolysis.
“The amount of power transferred through the solution is directly proportional to the mass of any substance deposited or dissolved at any electrode during electrolysis.”
“The amount of any substance participating in a reaction at an electrode is directly proportional to the quantity of electricity transmitted through the solution,” according to another definition.
If Wg of any material is deposited or dissolved when Q coulombs of electricity are transmitted, then WQ is the result.
However, Q=It
As a result, WI it
Or W=Z I t...
Where Z is the substance's electrochemical equivalent.
If I equal 1 unit (for example, 1 ampere) and t equals 1 unit (say, 1 second). When a current of one ampere is passed for one second, Z=W, i.e., the electrochemical equivalent of a substance equals the mass of the substance deposited or dissolved.
Electrolysis's main product.
The principal product of electrolysis is the substance generated directly by the current at the electrode surface during electrolysis. For instance, consider the electrode response.
Cu2+ + 2e– → Cu,
Copper (Cu) is the primary product.
Q2) Explain Faraday’s – First and Second Law of Electrolysis.
A2) Faraday’s – First Law of Electrolysis
It's one of the fundamental principles of electrolysis. It asserts that the amount of chemical reaction that takes place at any electrode under the influence of electrical energy is proportionate to the amount of electricity that passes through the electrolyte during electrolysis.
Faraday’s – Second Law of Electrolysis
When the same amount of power is passed through the electrolytic solution during electrolysis, a number of distinct compounds are freed in proportion to their chemical equivalent weights (Equivalent weight is defined as the ratio of the atomic mass of metal and the number of electrons required for reducing the cation).
We can derive from these electrolysis equations that the quantity of electricity required for oxidation-reduction is dependent on the electrode reaction's stoichiometry.
As an example,
++e
One mole of electrons is required for the reduction of one mole of sodium ions, as can be seen. We know that the charge on a single electron is 1.6021 10-19 C. As a result, the charge on one mole of electrons is:

This quantity of electricity is indicated by F and is defined as the charge carried per unit mole of electrons.
The nature of the material being electrolyzed and the type of electrodes employed determine the outcome of an electrolytic reaction. An inert electrode, such as platinum or gold, does not participate in the chemical reaction and merely serves as a source or sink for electrons. A reactive electrode, on the other hand, is a participant in the reaction.
As a result, in the case of reactive and inert electrodes, various electrolytic products are formed. The products of electrolysis are affected by the oxidising and reducing species present in the electrolytic cell, as well as their standard electrode potential.
Q3) Define Electrode Potential Definition and Explain its Significance.
A3) In an electrochemical cell, the standard electrode potential occurs when the temperature is 298K, the pressure is 1atm, and the concentration is 1M. The symbol Eocell denotes a cell's standard electrode potential.
Significance of Standard Electrode Potential
1. Redox reactions, which are made up of two half-reactions, constitute the foundation of all electrochemical cells.
2. The anode undergoes the oxidation half-reaction, which results in the loss of electrons.
3. At the cathode, a reduction reaction occurs, resulting in an electron gain. Electrons move from the anode to the cathode in this way.
4. The difference in the unique potentials of each electrode causes the electric potential to arise between the anode and the cathode (which are dipped in their respective electrolytes).
5. A voltmeter can be used to determine the cell potential of an electrochemical cell. A half-individual cell's potential, on the other hand, cannot be reliably quantified on its own.
6. It's also worth noting that this potential can alter as pressure, temperature, or concentration changes.
7. Standard electrode potential is required to achieve the individual reduction potential of a half-cell.
8. It is measured with the use of a conventional hydrogen electrode as a reference electrode (abbreviated to SHE). SHE has a 0-volt electrode potential.
9. By connecting an electrode to the SHE and measuring the cell potential of the resulting galvanic cell, the standard electrode potential of an electrode can be determined.
10. An electrode's oxidation potential is the inverse of its reduction potential. As a result, an electrode's standard electrode potential is characterised by its standard reduction potential.
11. Good oxidising agents have high standard reduction potentials, whereas good reducing agents have low.
Ca+ has a standard electrode potential of -3.8V, while F2 has a standard electrode potential of +2.87V. This means that F2 is an excellent oxidizer, whereas Ca is a reducing agent.
Q4) What is Electrochemical cell notation?
A4) Remember that for both oxidation and reduction reactions, standard cell potentials may be calculated using potentials E0cell. A positive cell potential implies that the reaction is proceeding spontaneously in the direction specified. A reaction with a negative cell potential, on the other hand, continues in the opposite direction spontaneously.
E0 reduction + E0 oxidation = Eo cell
Voltaic or galvanic (spontaneous) cells are described using cell notations. This unique shorthand describes the reaction circumstances (pressure, temperature, concentration, and so on), as well as the anode, cathode, and electrode components.
Remember that oxidation occurs at the anode and reduction occurs at the cathode. Electrons move from the anode to the cathode when the anode and cathode are connected by a wire.
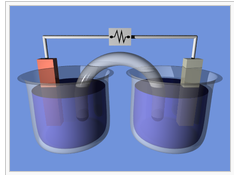
A typical galvanic cell: A typical arrangement of half-cells linked to form a galvanic cell.
Using the arrangement of components, let’s put a cell together.
One beaker contains 0.15 M Cd(NO3)2 and a Cd metal electrode. The other beaker contains 0.20 M AgNO3 and a Ag metal electrode. The net ionic equation for the reaction is written:
2Ag+ (aq) + Cd (s) Cd{2+} (aq) + 2Ag (s)
In the reaction, the silver ion is reduced by gaining an electron, and solid Ag is the cathode. The cadmium is oxidized by losing electrons, and solid Cd is the anode.
The anode reaction is:
Cd (s) Cd{2+} (aq) + 2e-
The cathode reaction is:
2Ag+ (aq) + 2e- 2Ag (s)
Q5) What is Galvanic Cell? Explain Its Principle.
A5) A galvanic cell or voltaic cell is an electrochemical cell that converts the chemical energy of spontaneous redox reactions into electrical energy.
Galvanic cell is a type of electrochemical cell. A voltaic cell is an electrochemical cell that generates electricity through chemical processes.
Let's have a look at how a voltaic or galvanic cell is made.
Electrons are transferred from one species to another in oxidation-reduction processes. If a reaction occurs spontaneously, energy is released. As a result, the liberated energy is put to good use. To deal with this energy, the reaction must be divided into two half-reactions: oxidation and reduction. The reactions are put into two distinct containers with wire in order to move the electrons from one end to the other. This results in the formation of a voltaic cell.
Principle of Galvanic (Voltaic) Cell
The Gibbs energy of spontaneous redox reaction in the voltaic cell is primarily responsible for the electric work done by a galvanic cell. It consists of two half cells and a salt bridge in most cases. A metallic electrode immersed in an electrolyte is also included in each half cell. With the help of metallic wires, these two half-cells are externally connected to a voltmeter and a switch. A salt bridge is not always necessary when both electrodes are submerged in the same electrolyte.
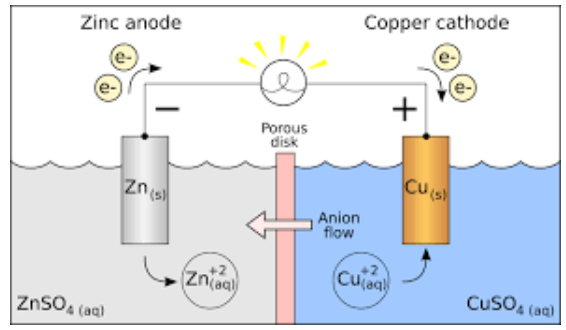
Galvanic Cell (Voltaic Cell) Diagram
Q6) Explain the Parts and Working of Galvanic Cell.
A6) Parts of Galvanic Cell
1. Anode - This electrode is where oxidation takes place.
2. Cathode - This electrode is where reduction takes place.
3. Salt bridge - A salt bridge contains the electrolytes needed to complete the circuit in a galvanic cell.
4. Half-cells – the reduction and oxidation reactions are compartmentalised.
5. External circuit - Allows electrons to travel freely between electrodes.
6. Load - A component of the circuit that relies on the flow of electrons to complete its function.
Working of Galvanic Cell
1. When an electrode is exposed to the electrolyte at the electrode-electrolyte interface in a galvanic cell, the atoms of the metal electrode tend to create ions in the electrolyte solution, leaving the electrons at the electrode behind. As a result, the metal electrode becomes negatively charged.
2. Metal ions in the electrolyte solution, on the other hand, have a tendency to settle on a metal electrode. As a result, the electrode becomes positively charged.
3. Charge separation is observable under equilibrium conditions, and the electrode can be positively or negatively charged depending on the inclinations of two opposing reactions. As a result, a potential difference between the electrode and the electrolyte develops.
4. The electrode potential is the difference in potential between the two electrodes.
5. The electrode that undergoes oxidation is known as the anode, while the electrode that undergoes reduction is known as the cathode.
6. The cathode has a positive potential in relation to the solution, whereas the anode has a negative potential in relation to the solution.
7. As a result, a potential difference occurs between the galvanic cell's two electrodes. The differential in potential is referred to as cell potential.
Cell potential is defined as the electromotive force of the galvanic cell when no current is extracted from it.
9. When the switch is turned on, electrons flow from the negative electrode to the positive electrode due to the potential difference.
Q7) What is an Electrochemical Cell?
A7) An electrochemical cell is a device that may either create electrical energy from chemical processes taking place inside it or use electrical energy supplied to it to aid chemical reactions taking place inside it. Chemical energy can be converted to electrical energy or vice versa using these devices. A conventional 1.5-volt cell, which is used to power numerous electrical items such as TV remotes and clocks, is an example of an electrochemical cell.
Galvanic cells or Voltaic cells are cells that can generate an electric current from chemical reactions that occur within them. Electrolytic cells, on the other hand, are cells that undergo chemical reactions when an electric current is delivered through them.
Below is a diagram that shows the many components of an electrochemical cell.
Cell Electrochemical
A cathode and an anode are the most common components of electrochemical cells. The essential characteristics of the cathode and anode are listed below.
Cathode | Anode |
Because electrons are spent here, it is denoted by a positive sign. | Because electrons are freed here, it is denoted by a negative sign. |
An electrochemical cell's cathode undergoes a reduction reaction. | Here, an oxidation reaction takes place. |
The cathode attracts electrons. | Electrons are ejected from the anode. |
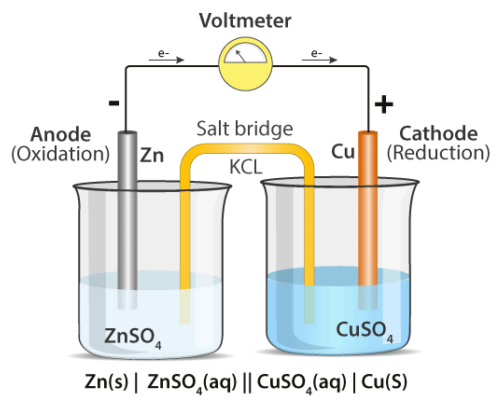
When signifying an electrochemical cell, the cathode must be depicted on the right-hand side, whilst the anode must be represented on the left-hand side.
Q8) Differences between Galvanic cells and electrolytic cells.
A8)
Galvanic Cell / Voltaic Cell | Electrolytic Cell |
In these electrochemical cells, chemical energy is converted to electrical energy. | In these cells, electrical energy is converted to chemical energy. |
The redox reactions that occur in these cells are entirely spontaneous. | The redox reactions in these cells require an energy input to proceed, indicating that they are non-spontaneous. |
The anode is negatively charged while the cathode is positively charged in these electrochemical cells. | A positively charged anode and a negatively charged cathode are used in these cells. |
The species that undergoes oxidation is where the electrons come from. | Electrons are emitted from a source outside of the body (such as a battery). |
Q9) Explain Reversible Process in Details.
A9) Every day, we witness several changes such as water boiling, iron rusting, ice melting, paper burning, and so on. In all of these processes, we notice that the system in question transitions from an initial state to a final state in which the system absorbs some heat from the environment and performs some work W on the environment. Now, how many of these systems can the system and its environment be restored to their original state? With popular instances like rusting and fermentation, we may conclude that it is not possible in the vast majority of circumstances. We will study about reversible and irreversible processes in this part.
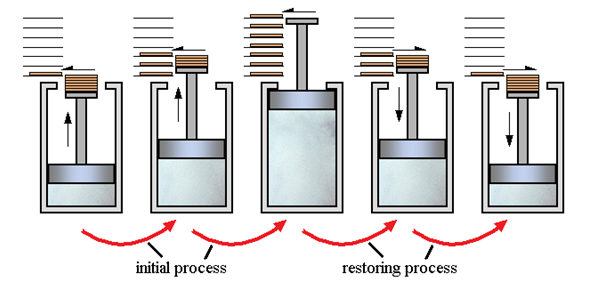
Reversible and Irreversible Processes
A thermodynamic process (state I state f) is considered to be reversible if it can be reversed so that the system and its surroundings return to their original states with no further changes in the universe. As we all know, there are no such things as reversible processes in reality. Reversible processes can thus be simply characterised as idealizations or models of real processes, based on which the system or device's boundaries have to be specified. They assist us in determining the maximum efficiency a system can deliver under ideal operating conditions, as well as the target design that can be established.
Q10) Give Examples of Reversible Process and Explain its Reversible Changes.
A10) We've included a few instances of reversible processes below:
1. Springs' extension
2. Adiabatic compression or expansion of gases at a slow rate
3. The process of electrolysis (with no resistance in the electrolyte)
4. The motion of solids without friction
5. Gases are compressed or expanded at a slow, isothermal rate.
Reversible Changes
Reversible modifications are any alterations that can be reversed or are a temporary conversion. Reversible reactions are those that are reversible in nature. A material is transformed into a different form in this reaction, but no new chemical is produced. Melting, boiling, evaporation, freezing, condensation, and disintegration are examples of reversible transformations. Melting wax, freezing ice, and boiling water that evaporates as steam and condenses back to water are a few examples.
Reactions are the result of the interaction of two or more reactants to form a product (s). A two-way arrow () connects the reactants and products created in a reversible process. This indicates that reactants can be recovered from products.
Consider the following reaction:
A +B ⇌ C + D
A and B are two reactants that combine to form C and D. The reaction is reversible, as indicated by the two-headed arrow, and the reactants A and B can be recovered from C and D.
Q11) What are Irreversible Processes? Give Examples
A11) An irreversible process is one in which the system and its surroundings do not revert to their original state after the process has been started. Consider the case of an automotive engine that has travelled a certain distance using a certain amount of fuel. The fuel burns to generate energy to the engine, converting itself to smoke and heat energy in the process. We will never be able to recover the energy lost by the fuel, nor will we ever be able to return to its original state. The irreversibility of a process is caused by a number of reasons, including:
1. Friction that turns the fuel's energy into heat energy.
2. The unregulated expansion of the fluid, which prevents the fuel from returning to its previous state. The reversal of heat transfer through a finite temperature is not feasible because the forward process is spontaneous in this scenario.
3. Intermixing of two different substances that cannot be separated since the process is spontaneous in nature, and the opposite is not possible.
As a result, depending on their ability to return to their original form from their final state, certain processes are reversible and others are irreversible.
Examples of Irreversible Processes
Irreversible Processes include the following:
1. Frictional relative motion
2. Suffocation
3. The transport of heat
4. Dissemination
5. Flow of electricity through a resistance
Q12) What is Electromotive Force? Explain Its Formula.
A12) The electric potential generated by an electrochemical cell or by changing the magnetic field is known as electromotive force. Electromotive force is frequently abbreviated as EMF.
Energy is converted from one form to another using a generator or a battery. One terminal becomes positively charged, while the other becomes negatively charged in these devices. As a result, an electromotive force is work performed on a single unit of electric charge.
The electromagnetic flowmeter, which is based on Faraday's law, employs electromotive force.
Symbol for Electromotive Force
The electromotive force symbol is ε.
Electromotive Force Formula
Following is the formula for electromotive force:
ε = V + Ir
Where I. V is the cell's voltage and II. I is the circuit's current.
3. r is the cell's internal resistance.
The electromotive force (EVF) is the fourth force.
Q13) Difference between Electromotive Force and Potential Difference.
A13)
Electromotive Force | Potential Difference |
The work done on a unit charge is defined as EMF. | The energy dissipated when the unit charge passes through the components is defined as the potential difference. |
The EMF does not change. | The difference in potential is not constant. |
The EMF is unaffected by circuit resistance. | The resistance between the two sites during the measurement determines the potential difference. |
Electric, magnetic, and gravitational fields are all caused by EMF. | The only electric field is induced due to the potential difference. |
E is the symbol for it. | V is the symbol for it. |
Q14) Explain the Types of Electrochemical Cell.
A14) Galvanic Cell
Galvanic Cell is named after Italian scientist Luigi Galvani. A galvanic cell is a type of electrochemical cell that serves as the foundation for many others, including the Daniell cell. It is made up of two separate metallic conductors known as electrodes, each of which is immersed in its own ionic solution. Each of these configurations is half of a cell. A half cell cannot generate a potential difference by itself. However, when they are combined, they can make a significant difference. A salt bridge is utilised to chemically connect the two cells. It transfers electrons from the electron-rich half cell to the electron-deficient half cell in the needed amount.
Let's look at the Daniell cell theory and develop the Nernst equation for it to make things clearer.
Daniell Cell
The galvanic cell has been adapted into the Daniell cell. It's made up of zinc and copper electrodes that have been immersed in zinc and copper sulphate solutions, respectively. A salt bridge is used to connect two half cells together. The zinc electrode serves as the anode, while copper serves as the cathode.
When compared to the copper metal, the zinc metal comes out on top in the electrochemical series due to its larger oxidation potential. As a result of the oxidation of zinc, two electrons and a zinc ion are produced. When compared to the other electrode, this electrode gets a negative potential due to the release of electrons. It's referred to as an anode.
Copper, on the other hand, is reduced due to its higher reduction potential. The copper ion in the copper half cell solution absorbs two electrons from the electrode and transforms into copper metal, which is then deposited in the electrode. Because this electrode consumes electrons, we refer to it as a positive electrode, and we refer to it as a cathode.
The following is a diagram of the anode reaction:
Zn(s) → Zn2+ (aq) + 2e–
The cathode reaction is represented as follows:
Cu2+ (aq) +2e– → Cu(s)
The combined cell reaction or overall cell reaction is as follows:
Zn(s) + Cu2+(aq) → Zn2+ (aq) + Cu(s)
Amount of Discount on Issue of Debenture
Q15) Explain Entropy of electrochemical cell.
A15) The Gibbs function is related to entropy through its temperature dependence

A similar relationship can be derived for the temperature variance of Eo.

Consider the following data for the Daniel cell (Buckbeei, Surdzial, & Metz, 1969) which is defined by the following reaction
Zn(s)+Cu2+(aq) ⇌Zn2+(aq)+Cu(s)
T (°C) | 0 | 10 | 20 | 25 | 30 | 40 |
Eo (V) | 1.1028 | 1.0971 | 1.0929 | 1.0913 | 1.0901 | 1.0887 |
From a fit of the data to a quadratic function, the temperature dependence of

Is easily established.
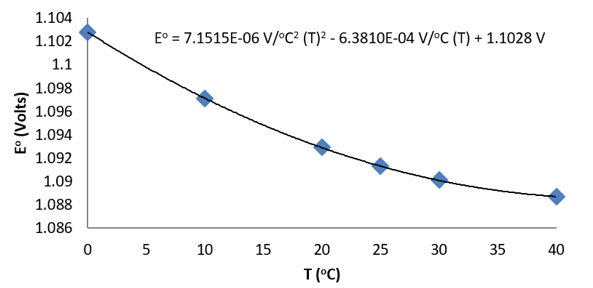
Figure: Temperature dependence of the cell potential for a Daniel cell.
The quadratic fit to the data results in

So, at 25 °C,
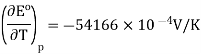
Noting that KK can be substituted for °C°C since in difference they have the same magnitude. So, the entropy change is calculated (Equation 10.4.2) is

Because

The standard entropy changes for the Daniel cell reaction at 25 °C is

It is the negative entropy change that leads to an increase in standard cell potential at lower temperatures. For a reaction such as

The standard cell potential drops with decreasing temperature due to a considerable increase in entropy (due to the generation of a gas-phase product). Because this is the reaction that most automobile batteries use, it explains why starting a car on a chilly winter morning can be difficult. Bratsch reports and discusses the temperature dependence of numerous typical cell potentials (Bratsch, 1989).
Q16) What is Equilibrium constants?
A16) It relates the amounts of reactants and products at equilibrium for a chemical reaction. For a general chemical reaction occurring in solution,
AA + bB ⇄ cC + dD
The equilibrium constant, also known as Keq, is defined by the following expression:

Where [A] is the equilibrium molar concentration of species A, and so on. In the expression for Keq, the coefficients a, b, c, and d in the chemical equation become exponents. The Keq is a numerical value that represents a distinctive numerical value for a specific reaction at a certain temperature; each chemical reaction has its unique Keq. In an equilibrium chemical reaction, the concentrations of each reactant and product are connected; the concentrations cannot be arbitrary numbers, but they do depend on each other. The concentrations of every product (whatever many products there are) are in the numerator of the expression for Keq, while the concentrations of every reactant are in the denominator, resulting in the common products over reactants definition for Keq.
Consider the following scenario. Assume we've reached this point of equilibrium:
A ⇄ B
There is one reactant, one product, and the coefficients on each are just 1 (assumed, not written). The Keq expression for this equilibrium is

(On each concentration, the exponents of one are understood.) Assume that the Keq value for this chemical reaction is 2.0. If [B] = 4.0 M, then [A] must equal 2.0 M for the fraction's value to be 2.0:
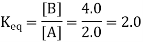
The units are assumed to be M and are omitted from the Keq statement by convention. Assume [B] is 6.0 M. [A] would have to be 3.0 M at equilibrium for the Keq value to remain constant (it is, after all, named the equilibrium constant):
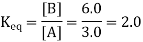
The reaction would not be at equilibrium if [A] was not equal to 3.0 M, and a net reaction would proceed until the ratio reached 2.0. The reaction will be at equilibrium at that point, and any net change will stop. (Remember, however, that chemical equilibrium is dynamic, thus forward and reverse reactions do not halt.)
With more sophisticated formulas for the Keq, the problem remains the same; only the mathematics becomes more difficult. In general, the missing concentration may be determined given a value for the Keq and all but one concentration at equilibrium.
Q17) Give Characteristics of Equilibrium Constant.
A17)
- It's reaction-specific, and it's fixed at a constant temperature.
- To keep the value of the equilibrium constant, a catalyst changes the rate of forward and backward reactions in the same way.
- Changes in concentration, pressure, temperature, and inert gases may influence the equilibrium, favouring one of the two reactions but not the equilibrium constant.
Is related to the standard free energy as, △G0 = -RT ln Kequ.
- Kequ has different values at different temperatures for the same reversible process.
- The reverse equilibrium's equilibrium constant is the reciprocal of the original equilibrium, i.e., Krev = 1/Kequ.
- If the stoichiometry of the equilibrium reaction is modified, the power of the equilibrium constant is likewise modified by the same amount.
- If K is the equilibrium constant for the reaction A + B C + D, then K3 is the equilibrium constant for the reaction 3A = 3B 3C + 3D.
- If there are numerous stepwise equilibria leading to the end products, the net equilibrium constant is the product of each stepwise equilibrium constant. As a result, K = K1 K2 K3 is the net equilibrium constant.
- Equilibrium reactions with a common product that occur simultaneously. The reaction's equilibrium constant does not change. The product concentrations will be reduced due to the larger concentration of the common product.
Q18) What is Hydrogen potential and pH-value?
A18) The hydrogen potential is directly proportional to the activity or rather the concentration of hydronium ions in the solution, and hence to the pH value. The negative decadic logarithm of the hydrogen ions activity is used to get the pH value. The product of concentration and activity coefficient is activity. In diluted solutions, the latter can only be approximated. As a result, measuring the pH-value according to its definition can only be done roughly.

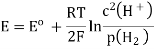
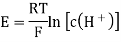
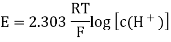
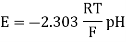



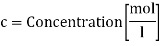
Saturated vapour pressure [bar]



Hydrogen potential and hydrogen ions concentration and thereby the pH-value are directly depending on each other.
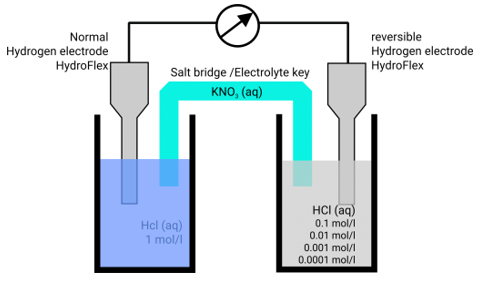
In hydrochloric acid, a concentration chain of two platinum hydrogen half cells
A concentration chain or rather a concentration cell is formed when two platinum hydrogen electrodes, one immersed in an acid with a proton’s concentration of 1 mol/l, pH = 0, (normal hydrogen electrode, NHE) and the other in a solution with an unknown proton’s concentration (reversible hydrogen electrode, RHE), are connected by a salt bridge.
The potential of the spontaneous occurring reaction sets as soon as two half cells are touched and the circuit is closed. The hydrogen ions are converted to hydrogen due to the increased proton concentration in the left half-cell. As a result of the removal of electrons from the electrode, it now has a higher positive potential than the electrode in the right half-cell. As a result, the decrease occurs in the left half-cell. The positive pole of the galvanic cell is that cell, which is comparable to the cathode. Hydrogen is oxidised to hydrogen ions in the right half-cell. The electrode on the right has a lower voltage than the electrode on the left (negative pole). The oxidation takes occurs in the right half-cell, making the right half-cell equivalent to the anode. The electrons freely flow from the negative pole to the positive pole, or from the anode to the cathode in this case (galvanic cell). From the diluted to the concentrated cell, electrons travel from the right to the left.
Q19) Write short note on Reduction of Quinones.
A19) A characteristic and important reaction of quinones is reduction to the corresponding arenediols. The reduction products of 1,4-quinones are called hydroquinone’s:
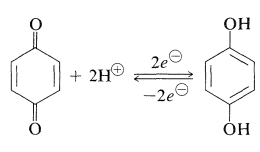
Electrochemical reduction and a number of reducing chemicals can be used to produce reduction (metals in aqueous acid, catalytic hydrogenation). These reductions are rare among organic reactions in that they are fast and reversible enough to provide easily repeatable electrode potentials in an electrolytic cell. The square of the hydrogen-ion concentration determines the position of the 1,4-benzenediol-1,4-benzenedione equilibrium (Equation 26-2). As a result, the electrode potential is pH sensitive; a change of one unit of pH in water affects the electrode potential by 0.059V0.059V. The half-cell potential created by this equilibrium was commonly employed to calculate pH values of aqueous solutions before the invention of the glass-electrode pH metre. Because the quinone interacts permanently with alkali over pH 9, the procedure isn't particularly useful above that point.
The link between half-cell reduction potentials and quinone structures has been studied extensively. The potentials are greatest when the resonance stabilisation associated with the creation of the aromatic ring is greatest, as one might predict.
When hydroquinone and quinone alcohol solutions are combined, a brown-red hue appears and a green-black 1:1 complex known as quinhydrone crystallises. The diol acts as an electron donor, and the dione acts as an electron acceptor in this charge-transfer complex (Section 24-6C). Quinhydrone is a water-insoluble compound that readily dissociates into its constituents in solution.
Two electrons are required for the reduction of a quinone, and these electrons can be transmitted simultaneously or one at a time. A single-electron transfer produces a semiquinone, which has both a negative charge and an odd electron (a radical anion):
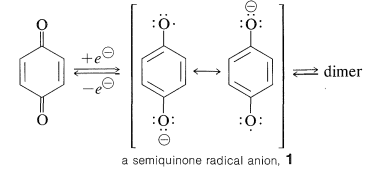
A variety of approaches have been used to demonstrate that electrolytic reduction of quinones produces rather stable semiquinone radicals. Reversible dimerization processes create peroxides from certain semiquinone radicals.
Mild oxidation of NN, NN, N′N′, N′N′-tetramethyl-1,4-benzenediamine produces a semiquinone cation-radical that is highly stable. "Wurster's Blue" is the name given to the cation, which can be isolated as a brilliant-blue perchlorate salt:
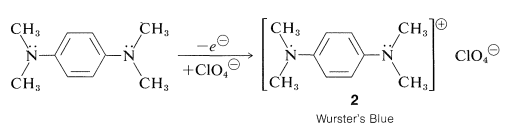
Q20) Explain the Principles of the Glass-Electrode Method.
A20) The known pH of a reference solution is calculated using the glass-electrode method, which involves utilising two electrodes, a glass electrode and a reference electrode, and measuring the voltage (difference in potential) generated between the two electrodes. The electromotive force is proportional to the difference in pH between the liquids inside and outside the thin glass barrier. The electrode membrane is the name given to this thin membrane. When the temperature of the solution is 30 °C and the pH within is 1 higher than the pH outside, roughly 60 mV of electromotive force is generated.
The pH of the liquid inside the glass electrode is usually about 7. When the electromotive force generated at the electrode membrane is measured, the pH of the sample can be calculated.
When measuring the electromotive force generated at the electrode membrane of a glass electrode, a second electrode is required. The reference electrode is the other electrode that is used in conjunction with the glass electrode. The potential of the reference electrode must be exceedingly steady. As a result, the liquid connection is equipped with a pinhole or a ceramic substance.
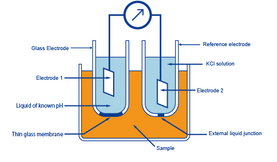
To put it another way, a glass electrode is used to generate precise electromotive force as a result of a pH difference. And a reference electrode is designed to prevent electromotive force from being caused by a pH variation.
On the market, there are a variety of pH glass electrodes. Because each pH glass electrode has its own set of characteristics, it's important to pick the right one for the job.
Q21) What different kinds of pH glass electrodes are available?
A21) Due to the process of taking pH measurements, the glass membrane of a pH glass electrode changes over time. Alkali ions dissolve from the glass surface when exposed to water, and the oxide groups of the silicate become OH groups, forming a source layer. To hydrogen ions, this source layer seems to be an ion exchanger. There is a consistent balance between the sample solution and the glass surface using a unique pH glass electrode membrane, which is only reliant on the hydrogen ion concentration in the solution and the source layer.
Because pH glass electrodes have a wide range of capabilities, a variety of membrane glasses are required to get precise and consistent pH measurements in all applications. L-, H-, S-, A-, and N- are the five various varieties of pH glass electrodes offered by SI Analytics. The following are the key characteristics of these pH glass electrodes:
1. L type pH glass electrode: Wide application range, low impedance, accurate and quick response times over a wide temperature range.
2. H type pH glass electrode: Designed for greater temperatures (up to 135°F) and severe pH values, with exceptional accuracy in the stronger alkaline range (Na+).
3. S type pH glass electrode: Tolerates rapid temperature changes and gives consistent measurement values in hot alkali solutions with a quick response time.
4. A pH glass electrode of the following type: Drinking water, surface water, sewage, and general applications all benefit from quick response times.
5. N type pH glass electrode: Useful for the entire pH range and practically all types of samples at normal temperatures.
Q22) What different kinds of pH Glass Electrode Membrane Shapes are available?
A22) Glass pH electrodes come in a variety of membrane designs. Each pH glass electrode is made to fit a specific pH measurement application.
1. Sphere pH glass electrode membrane: Excellent consistency, low resistance due to high surface area, and suitable for most applications.
2. Dome pH glass electrode membrane: They have a higher resistance, are shock-proof, and are easy to clean, making them ideal for automated cleaning systems.
3. Flat pH glass electrode membrane: High resistance, shock-proof, easy to clean, and suitable for surface pH measurements.
4. Cylinder pH glass electrode membrane: Medium resistance, shock-proof, and suitable for a wide range of applications, including fermentation.
5. Spear pH glass electrode membrane: High resistance, ideal for penetrating semi-solid media and measuring systems with automated ultrasonic cleaning.
6. Cone pH glass electrode membrane: Sturdy, smooth, simple to clean, and generally applicable.
The type and shape of the pH glass electrode membrane glass are critical to making reliable pH measurements. The pH glass electrodes' findings and features are influenced by the glass composition, manufacturing methods, and shape. Only the greatest pH glass electrode will give you with the highest level of accuracy and dependability.
Q23) Give Example of Galvanic Cell.
A23) More than a century ago, electrochemical or galvanic cells were introduced as a technique for researching the thermodynamic features of fused salts. A galvanic cell, such as Daniel's cell, turns chemical energy into electrical energy. Copper ions are reduced at the cathode in Daniel's cell, while zinc is oxidised at the anode.
Daniel cell reactions at the cathode and anode are as follows:
At cathode: Cu 2+ + 2e– → Cu
At anode: Zn → Zn2+ + 2e–