Unit - 3
Catalysis by Organometallic Compounds
Q1) Write a short note on Alkene metathesis.
A1) The use of alkene metathesis reactions to make unsaturated olefinic compounds and their polymeric counterparts is gaining popularity. Depending on the reaction conditions of the metathesis reaction, a metal carbene intermediate reacts with olefins to produce various olefinic compounds or even unsaturated olefinic polymers. Metathesis is an unusual reaction in which a C=C bond is broken and a new unsaturated olefin is formed during catalysis.

Only a few metalcarbene catalysts have been found to be functional group tolerant, despite the fact that a large number of metalcarbene catalysts have been developed for the metathesis reaction. As a result, developing functional group tolerant catalysts has been a crucial step in broadening the utility of the metathesis reaction. Early-transition metal based carbene catalysts, such as those based on Ti, are highly oxophilic and thus intolerant of functional groups in this regard. The more electron-rich Mo and W-based catalysts, on the other hand, are classified as intermediate. Finally, Ru catalysts based on late-transition metals are found to be extremely tolerant of functional groups while still exhibiting high reactivity toward olefinic bonds. The Grubb's Ru catalyst, which is simple to use, and the Schrock's Mo catalyst, which has a high activity, are noteworthy in this context.
The term "metathesis" refers to a group of reactions that all involve the "cutting and stitching" of olefinic bonds to produce various unsaturated products. Because the olefinic ends are exchanged when two different olefin substrates are used, this reaction is known as "cross metathesis."
In systems where the ring strain in the final product is not too high, the metathesis reactions can even extend to conjugated dienes, which can undergo Ring Closing Metathesis (RCM). Ring Opening Metathesis (ROM) is the reversal of Ring Closing Metathesis (RCM), and it's usually preferred when there's a lot of C2H4.
The Acyclic Diene Metathesis (ADMET) and the Ring Opening Metathesis Polymerization (ROMP), in which the relief of cycloalkene ring-strain drives the polymerization reaction forward, are two common metathesis variants used in the production of polymers. Both of these reactions produce living long chain polymers, making them useful for making block copolymers (AAABBBB) n.
Q2) Explain Designing organometallic compounds for catalysis.
A2) Bioorganometallic chemistry is an area of research that is rapidly evolving. Organometallic compounds have recently provided a rich platform for the development of efficient catalysts for olefin metathesis and transfer hydrogenation, for example. Thermodynamics and kinetics of ligand substitution and redox reactions of metal ions, particularly RuII, are controlled using electronic and steric effects. Is it possible to design targeted organometallic drugs that incorporate similar features. Through the incorporation of outer-sphere recognition of targets and controlled activation features based on ligand substitution as well as metal- and ligand-based redox processes, such complexes have the potential to provide novel drug action mechanisms. The 6-arene, 5-cyclopentadienyl sandwich, and half-sandwich complexes of FeII, RuII, OsII, and IrIII with promising activity against cancer, malaria, and other conditions are the focus of this paper.
Catalysis is an important point to remember. Catalysis frequently involves organometallic complexes. Hydrogenation, hydrosilylation, hydrocyanation, olefin metathesis, alkene polymerization, alkene oligomerization, hydrocarboxylation, methanol carbonylation, and hydroformylation are some of the most commonly used industrial processes.
Homogeneous catalysts, heterogeneous catalysts, and biocatalysts are the three types of catalysts and catalytic reactions (usually called enzymes).
Q3) Explain mechanism of Hydrogenation - Catalytic Cycle.
A3) Wilkinson's catalyst is, in fact, a pre-catalyst that is converted to an active form by removing one triphenylphosphine ligand before entering the catalytic cycle. The vacant site is usually filled by the solvent molecule.
Initially, the catalyst uses an oxidative addition mechanism to activate the molecular dihydrogen, resulting in a dihydrido complex with 18 valence electrons. Rh's oxidation state is now +3. In the next step, the formed dihydrido complex binds to the olefin, releasing the solvent or PPh3 ligand in the process. This path is referred to as the dihydride path because dihydrogen activation occurs before olefin addition.
At the double bond, one of the hydrogens is now inserted migratorily. The Rate Determining Step is a slow step (RDS).
An irreversible reductive elimination step completes the catalytic cycle by rapidly releasing the alkane. The catalyst is regenerated and the oxidation state of Rh is reduced to +1.
* When too much PPh3 is added, the rate of reaction slows down, indicating that one of the PPh3 ligands dissociates before dihydrogen activation.
*Strong -acids, such as ethylene, are thought to act as poisons because they bind tightly to the electron-rich Rh metal centre and prevent hydrogenation.
Q4) Describe the Selective Hydrogenation by Wilkinson’s Catalyst.
A4) Wilkinson's catalyst can be used to achieve selective hydrogenations.
* Double bonds that are less substituted and sterically less hindered are selectively hydrogenated.
* Exocyclic double bonds are selectively hydrogenated over endocyclic double bonds.
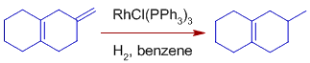
* Cis alkenes decompose more quickly than trans alkenes.
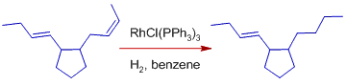
* Over conjugated dienes, isolated double bonds are rapidly hydrogenated.

* In comparison to terminal alkenes, terminal alkynes hydrogenate faster. By using acidic alcoholic co-solvents, the selectivity can be increased.

*C=O, C=N, NO2, Aryl, CO2R, and other functional groups are unaffected. Wilkinson's catalyst's compatibility with polar multiple bonds suggests that the metal hydride bond is mostly covalent.

Note that sterically unhindered aldehyde groups are susceptible to decarbonylation, making the catalyst ineffective as well.
* Hydrogenation occurs more quickly on unsaturated substrates with polar functionality. It could be due to the polar functional group's assistance in facilitating olefin coordination to the catalyst.
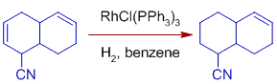
Q5) State the Stereochemistry of Wilkinson’s Hydrogenation.
A5) Wilkinson's catalyst catalyses stereospecific syn hydrometallation of multiple bonds followed by stereospecific reductive elimination in hydrogenation reactions. As a result, syn addition products result from hydrogenation of olefins or alkynes.
For example, the diastereoselective hydrogenation of Maleic or Fumaric acid with D2 in the presence of Wilkinson's catalyst. Maleic acid is hydrogenated with D2 to produce only the meso compound.
Whereas, with fumaric acid, a racemic mixture is formed.
Hence Wilkinson's hydrogenation is both stereospecific as well as stereoselective.
It is stereospecific in the sense that one stereo isomer produces only one stereo isomer. It's because of the mechanism's stereotypical nature.
It is stereoselective, however, because only one diastereomer is formed as the major product. Other mechanisms that operate under reaction conditions also contribute to this.
When alkynes are hydrogenated, the major products are cis alkenes.
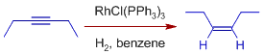
Wilkinson's hydrogenation has diastereoselectivity, as evidenced by the formation of an endo product as the major product with the bicyclic system below. The catalyst binds to the double bond on the bicyclic system's least hindered exo face, followed by the addition of two hydrogen atoms in syn.
Q6) State the Properties of Wilkinson’s Catalyst.
A6)
1. Physical Properties
Wilkinson's catalyst has a molecular mass of 925.22 g/mole.
It has a melting point of 518 to 523 degrees Celsius.
Water does not dissolve it. However, it is soluble in benzene and tetrahydrofuran, which are hydrocarbon-based solvents.
2. Chemical Properties
Wilkinson's catalyst is designed with a square planar coordination geometry.
RhCl(CO)(PPh3)2 is formed when it reacts with carbon monoxide.
When mixed with benzene, this coordination compound dimerizes. [RhCl(PPh3)2] is the chemical formula for the dimer's chemical composition. 2
• Homogeneous Catalysis
"When added to a reaction mixture, a catalyst changes the rate at which the system achieves equilibrium without undergoing a permanent chemical change." (Penguin Dictionary of Chemistry, Sharp, 1981) A catalyst speeds up the time it takes for the system to reach equilibrium, but it doesn't change the equilibrium because it also speeds up the back reaction. It is also necessary to define the following terms:
· A compound or complex that undergoes a reaction to produce the active catalyst.
· An auto catalyst is a reaction product that also serves as a catalyst in the same reaction.
· A compound that slows or stops a reaction is known as an inhibitor.
· TON = moles of product per mole of catalyst; substrate = molecule on which the catalyst acts; percent conversion = percent of the substrate converted to product; turnover number (TON) = moles of product per mole of catalyst (unitless)
· TOF = moles of product per mole of catalyst per hour (units are h-1)
A homogeneous catalyst is one in which the catalyst and the substrate are both in the same phase. An organic molecule, a salt, or a transition metal complex could all serve as homogeneous catalysts. The catalyst does not require a homogeneous solution. Homogeneous hydrogenation, for example, has two phases: hydrogen in the gas phase and a liquid phase containing the catalyst and the substrate (an olefin). The catalyst is a homogeneous catalyst, despite the fact that the system is technically heterogeneous.
Despite the fact that heterogeneous catalysts have long been the industry standard, homogeneous catalysts have a number of advantages (Table 1). Many industrial processes rely on them.
Q7) Describe Homogeneous Hydrogenation.
A7) When it comes to homogeneous hydrogenation, hydride ligands are crucial. At room temperature, hydrogen gas (H2) does not react with alkenes. By forming hydride complexes, metal complexes activate hydrogen and transfer it to alkenes. Hydrogenolysis, oxidative addition of H2, or heterolytic activation can all be used to create hydride complexes.
The hydrogen from the metal to the alkene must also be transferred. Hydrogen atom transfer, the hydride pathway, and the unsaturated pathway are the three most common ways for this to happen. Only the order in which hydrogen and alkene bind to the metal differs in the latter two routes.
MH + R2C=CR2 M• + R2HC-CR2• H atom transfer (radical hydr.)
MH2 + R2C=CR2 M(H)2(R2C=CR2) ® M(H)(CR2CHR2) ® M + CHR2CHR2 hydride pathway
M(R2C=CR2) + H2 M(H)2(R2C=CR2) ® M(H)(CR2CHR2) ® M + CHR2CHR2 unsaturated pathway
Wilkinson and Coffey independently discovered chlorotris(triphenylphosphine) rhodium(I), now known as "Wilkinson's catalyst," in 1964. The direct reaction of rhodium chloride with triphenylphosphine is the most straightforward way to make this complex, though it does not work well with other phosphines.
This catalyst was the first to be able to rapidly hydrogenate unconjugated alkenes and alkynes at extremely mild conditions (1 atm of H2 and at room temperature). The hydrogenation is usually performed in benzene with a polar cosolvent like ethanol. The polar solvent might facilitate migratory insertion, which is rate limiting.
RhCl(PPh3)3
RCH=CHR + H2 RCH2CH2R
Although some olefins, such as ethylene and 1,3 butadiene, are slowly reduced under ambient conditions and can act as powerful competitive inhibitors, steric factors play the most important role in determining olefin reactivity.
Q8) What is Hydroformylation reaction?
A8) Hydroformylation, also known as the "oxo" process, is a Co or Rh catalysed reaction that produces value-added aldehydes by combining olefins with CO and H2.
Otto Roelen discovered the reaction in 1938, and it quickly grew in scope and scale in terms of aldehyde production around the world. The hydroformylation reaction was catalysed by metal hydride complexes based on rhodium, HRh(CO)(PPh3)3, and cobalt, HCo(CO)4.
Hydroformylation, also known as oxo synthesis or oxo process, is a widely used industrial process for producing aldehydes from alkenes that uses a homogeneously catalysed reaction. 1st. A formyl group (CHO) and a hydrogen atom are introduced into a carbon-carbon double bond in this chemical reaction. Since its inception in 1938, this process has continued to expand: in 1995, the production capacity was 6.6106 tonnes. It's crucial because the aldehydes that result are easily converted into a variety of secondary products. The resulting aldehydes, for example, are hydrogenated to produce alcohols, which are then used to make plasticizers or detergents. Hydroformylation is also used in specialty chemicals like fragrances and natural products. One of the most significant achievements of twentieth-century industrial chemistry is the development of hydroformylation, which originated in the German coal-based industry.
Q9) What is Fischer-Tropsch Synthesis?
A9) The well-known and established catalytic chemical process known as Fischer-Tropsch (FT) synthesis, named after the original German inventors Franz Fischer and Hans Tropsch in the 1920s, can be used to produce liquid transportation hydrocarbon fuels and a variety of other chemical products from syngas. FT synthesis provided the German war effort with the necessary liquid hydrocarbon fuels during WWII. Later, when faced with isolation during the apartheid era, South Africa turned to FT synthesis from coal gasification to meet a large portion of its hydrocarbon fuel and chemical requirements.
Since then, many refinements and adjustments to the technology have been made, including catalyst development and reactor design.
The technology is called coal-to-liquids (CTL) or gas-to-liquids (GTL) depending on the source of the syngas (GTL). Sasol's Sasolburg I and II plants are examples of current CTL plants in operation, while Shell's plant in Bintulu, Malaysia, is an example of a GTL FT process. Several world-class GTL and CTL plants are currently under construction or in operation in Nigeria, Qatar, and China, as well as in the United States, with Sasol's Lake Charles Gas-to-Liquid (GTL) and Ethane Cracker Complex in Louisiana recently announcing front-end engineering and design. A simplified block flow diagram of a process using FT synthesis. All supporting process technologies, such as coal handling and feed preparation, heat recovery, syngas clean up and conditioning, water-gas-shift, sulphur recovery, and so on, are included in the gasification island. The clean syngas from the gasification island is transported to the FT synthesis island, where it is converted into wax, hydrocarbon condensate, tail gas, and reaction water as primary products. The wax is then sent to an upgrading unit where it is chemically split into smaller molecular weight hydrocarbon liquids by hydrocracking in the presence of hydrogen. A hydrogen recovery unit is used to extract the required amount of hydrogen from either the tail gas or the feed syngas stream, as shown. Depending on the desired product mix, the reaction products, as well as those from the upgrading section, are fractionated into diesel, naphtha, and other light ends. A number of utility plants, including the power train, support the manufacturing facility.
Q10) Explain the term F-T Reactors.
A10) Due to the high exothermic nature of the Fischer-Tropsch reaction, heat removal is a critical consideration in the design of a commercial reactor. For FT synthesis, there are three different reactor designs that can be used:
• Fixed bed reactor
• Fluidized bed reactor
• Slurry bed reactor.
Commercial reactors of all three types are available. Lurgi and Ruhrchemie collaborated on the development of the multitubular fixed-bed reactors known as Arge reactors, which were commissioned in 1955. They were used by Sasol in their Low-Temperature FT Synthesis Process in Sasolburg to produce heavy FT liquid hydrocarbons and waxes, with the goal of producing liquid fuels. Slurry-bed reactors, which are considered the state-of-the-art technology for low temperature FT synthesis, are now being used to replace most, if not all, of these types of Arge reactors. Temperature control and conversion are improved in slurry-bed FT reactors. Other Fischer-Tropsch technology providers, such as Exxon and Shell, are also working on slurry-bed FT reactors.
Exxon and Shell, for example, are Fischer-Tropsch technology providers.
Fluidized-bed FT reactors were created to produce low molecular gaseous hydrocarbons and gasoline at high temperatures. It was first developed in a circulating mode, such as Sasol's Synthol reactors, but it has since been replaced by Advanced Synthol reactors, which are a fixed fluidized bed type of design. High throughput is a feature of these reactors.
What can synthesis gas be used for, then? It can be burned and heat exchanged in a gas turbine to produce steam and power a second turbine. Electricity can be generated by feeding the gas into a solid oxide fuel cell. We can also make fuels, chemicals, and materials out of synthesis gas. The production of synthetic hydrocarbons for transportation fuels is, in fact, the most common application of synthesis gas from coal. FT synthesis (Fischer Tropsch). This is what the company Sasol does most of the time in South Africa, and it was also one of the methods used by the Germans to generate liquid fuels during WWII; in fact, direct liquefaction was the primary method used to produce liquid fuels in Germany during the 1940s. It is not, however, the only method for converting gas into liquids. As noted in Lesson 4, the FT synthesis reaction can be presented by:
CO + nH2→(-CH2−)x+H2O
We take carbon atoms and turn them into alkanes with at least 20 carbon atoms. It's actually a polymerization procedure that adheres to polymerization statistics. A typical polymerization statistical function is depicted in Figure 8.9. The FT process will not yield a single pure alkane, and the products will be distributed. You'll need to adjust reaction variables like temperature, pressure, residence time, and the addition of a catalyst, as you would with any chemical reaction. We can theoretically make anything from methane to high molecular weight waxes by carefully selecting the variables (T, P, t, and catalyst). The goal is to increase the production of liquid transportation fuel.
Q11) What is Methanol Production?
A11) Methanol, CH3OH, can also be made with synthesis gas. Methanol production technology is fairly mature at the moment. The feedstock is usually natural gas, which is steam reformed to produce CO and hydrogen:
CH4 + H2O→CO + H2
Then methanol is synthesized by the reaction:
CO + 2H2→CH3OH
However, another methanol synthesis reaction allows for CO2 to be in the feed gas:
CO2 + 3H2→CH3OH + H2O
However, because water and methanol are infinitely soluble, a downstream step is required to separate the methanol from the water. In the methanol synthesis reactor, typical operating conditions are 5-10 MPa pressure, 250-270°C, and a copper/zinc catalyst. Because the reaction is highly exothermic, heat must be removed to keep it under control. The reactor has a shell and tube heat exchanger, similar to the FT reaction, where the coolant is circulated through the shell and catalyst particles are packed into the tubes through which the reactant/product liquids flow.
Q12) Explain the MTG process.
A12) Methanol is a substance that can be used to produce gasoline. The method employs a zeolite catalyst with pore sizes large enough to allow molecules as large as C10 to pass through. Because this process cannot produce larger molecules, a product is created that contains no carbon molecules larger than C10, which boils in the gasoline range. Aromatics and branched-chain alkanes are produced in this process, resulting in a gasoline with a very high octane rating. The only product is gasoline. The following reaction transforms methanol into dimethyl ether (a good diesel fuel):
2CH3OH→CH3OCH3 + H2O
The dimethyl ether is dehydrated further to the product hydrocarbons. The overall reaction is:
CH3OH→−(CH2)−n+H2O
Because the reaction is highly exothermic, the reactor and process must be designed to remove heat from the reaction. 330-400°C and 2.3 MPa are the conditions for this reaction. One scenario for combining all of these processes in a plant is as follows:
1. Existing natural gas-fueled methanol plants can be upgraded with an MTG unit (produce high octane gasoline).
2. Coal and/or biomass gasification and gas conditioning should be used in place of natural gas.
3. Synthol reactors are added in parallel (produce high cetane diesel).
4. Add a third section that generates electricity from synthesis gas using a solid oxide fuel cell.
The plant then produces gasoline, diesel, and electricity.
Q13) State the all the groups involved in the Qualitative Cation Analysis.
A13) Group 1: Insoluble Chlorides
Only Ag+, Pb2+, and Hg22+ form chlorides that precipitate from water; most metal chloride salts are water soluble. In a qualitative analysis, the first step is to add about 6 M HCl, which precipitates AgCl, PbCl2, and/or Hg2Cl2. These cations aren't present in significant amounts if no precipitate forms. Using filtration or centrifugation, the precipitate can be collected.
Group 2: Acid-Insoluble Sulfides
The acidic solution is then suffused with hydrogen sulphide (H2S). Under these acidic conditions, only metal ions that form insoluble sulphides, such as As3+, Bi3+, Cd2+, Cu2+, Hg2+, Sb3+, and Sn2+, precipitate as sulphide salts. Fe2+ and Zn2+, for example, are still in solution. Filtering or centrifugation is used to collect the precipitates once more.
Group 3: Base-Insoluble Sulfides (and Hydroxides)
The solution is now basicified with ammonia or NaOH, followed by the addition of (NH4)2S. Any remaining cations that would otherwise form insoluble hydroxides or sulphides are removed during this procedure. The sulphides of the divalent metal ions Co2+, Fe2+, Mn2+, Ni2+, and Zn2+ form, while the hydroxides of the trivalent metal ions Al3+ and Cr3+ form: Al(OH)3 and Cr(OH)3, respectively. Sulfide reduces Fe3+ to Fe2+, which precipitates as FeS in the presence of sulphide in the mixture.
Group 4: Insoluble Carbonates or Phosphates
Metal ions that form insoluble carbonates and phosphates are the next ions to be removed from solution. Insoluble carbonates precipitate and are collected when Na2CO3 is added to the basic solution left over after the precipitated metal ions are removed. The same metal ions precipitate as insoluble phosphates when (NH4)2HPO4 is added.
Group 5: Alkali Metals
All metal ions that form water-insoluble chlorides, sulphides, carbonates, or phosphates have been removed at this point. Alkali metals (Li+, Na+, K+, Rb+, and Cs+) and ammonium (NH4+) are the only common ions that might survive. To produce NH3, we take a second sample from the original solution and add a small amount of NaOH to neutralise the ammonium ion. (Because we added ammonium to the sample in earlier steps, we can't use the same sample we used for the first four groups.) A litmus paper test or the odour of any ammonia produced can be used to detect it.The sodium is detected using a flame test on another original sample, which results in a bright yellow colour. In flame tests, the other alkali metal ions give off distinct colours, allowing them to be distinguished even if only one is present.
Q14) Describe Group 5: Alkali Metals in brief.
A14) All metal ions that form water-insoluble chlorides, sulphides, carbonates, or phosphates have been removed at this point. Alkali metals (Li+, Na+, K+, Rb+, and Cs+) and ammonium (NH4+) are the only common ions that might survive. To produce NH3, we take a second sample from the original solution and add a small amount of NaOH to neutralise the ammonium ion. (Because we added ammonium to the sample in earlier steps, we can't use the same sample we used for the first four groups.) A litmus paper test or the odour of any ammonia produced can be used to detect it. The sodium is detected using a flame test on another original sample, which results in a bright yellow colour. In flame tests, the other alkali metal ions give off distinct colours, allowing them to be distinguished even if only one is present.
Additional techniques, such as forming complex ions, changing the pH of the solution, or raising the temperature to redissolve some of the solids, are used to separate metal ions that precipitate together. For example, group 1 cation precipitated metal chlorides, such as Ag+, Pb2+, and Hg22+, are all water insoluble. However, because PbCl2 is much more soluble in hot water than the other two chloride salts, adding water to the precipitate and heating the resulting slurry will dissolve any PbCl2. If Pb2+ was present in the original sample, isolating the solution and adding a small amount of Na2CrO4 solution to it will result in a bright yellow precipitate of PbCrO4.
A bright yellow precipitate of PbCrO4 forms when a small amount of Na2CrO4 solution is added to a sample containing Pb2+ ions in water.
Treatment of group 1 cation precipitates with aqueous ammonia, for example, will dissolve any AgCl because Ag+ forms a stable complex with ammonia: [Ag(NH3)2]+. In addition, Hg2Cl2Hg2Cl2.
Q15) What is Qualitative Analysis?
A15) Qualitative analysis is a method of Analytical chemistry that deals with the determination of elemental composition of inorganic salts. It is mainly concerned with the detection of ions in an aqueous solution of the salt.
The common procedure for testing any unknown sample is to make its solution and test this solution with various reagents for the ions present in it. Testing with various reagents gives characteristic reaction of certain ions, which may be a colour change, a solid formation or any other visible changes. There are separate procedures for detecting cations and anions, called the Cation Analysis and Anion Analysis.
Q16) State all the Preliminary Tests in Qualitative Analysis of Cations.
A16) Some preliminary tests need to be done before doing the analysis of cations.
(a) Physical Appearance: Colour and Smell
The colour, smell, and density of the unknown salt are all examined physically. The test isn't very accurate, but it does help identify some coloured cations. Some ions, such as ammonium, have a distinct smell that aids in their identification.
(b) Charcoal Cavity Test
This test is based on the fact that metallic carbonates decompose to produce corresponding oxides when heated in a charcoal cavity. In the cavity, the oxides show up as a coloured incrustation or residue. In some cases, the formed oxides are partially reduced to metal, resulting in metallic beads or scales.
(c) Cobalt Nitrate Test (Ash test)
The salts that leave white residue in the charcoal cavity test are subjected to this test. This test is based on the fact that metallic carbonates decompose to produce corresponding oxides when heated in a charcoal cavity.
The test is based on the fact that heating causes cobalt nitrate to decompose into cobalt oxide, CoO. This reacts with the metallic oxides in the charcoal cavity, which are present as a white residue, to produce coloured compounds.
A while residue of MgO is left behind when a magnesium salt undergoes a charcoal cavity test, for example. This forms a double salt with the formula MgO.CoO, which is pink in colour, after being treated with cobalt nitrate and then heated.
(d) Flame Test
Certain salts form chloride when they react with concentrated HCl, which is volatile in a non-luminous flame. The flame takes on the colour of their vapours. The presence of certain cations can be reliably determined by this colour. To carry out this test, a platinum wire is used to introduce the paste of the mixture with conc.HCl into the flame.
(e) Borax Bead Test
On heating, borax, Na2B4O7.10H2O, fuses and loses crystallisation water. It expands into a fluffy white porous mass, which then melts into a colourless liquid, resulting in a clear transparent glassy bead made of boric anhydride and sodium metaborate.
Boric anhydride is not a flammable substance. It forms a distinctive coloured bead of metal metaborate when it reacts with a coloured metallic salt. Metaborates in various oxidation states of metals are formed in those cases where different coloured beads are obtained in oxidising and reducing flames. Copper forms blue copper metaborate in an oxidising flame, for instance.
Q17) What is interfering radicals in salt analysis?
A17) Oxalate, tartrate, fluoride, borate, and phosphate are anionic radicals that interfere with DNA synthesis. They form a complex with ammonium chloride and ammonium hydroxide, both of which belong to the IIIrd gr group of reagents. In an alkaline medium, this results in incomplete precipitation of IIIrd group cations and premature precipitation of IVth and Vth group cations. Let's take a look at it and see if we can figure out what's going on. In an acidic environment, metals such as oxalate, tartrate, fluoride, borate, silicate, and phosphate are soluble.
Remember that the medium for the first and second analyses is acidic (diluted HCl), so they don't interfere. However, the third group's reagents, ammonium chloride and ammonium sulphide, cause the medium to become alkaline. Interfering radicals interfere with the solubility product of cations, causing their precipitation to occur prematurely or incompletely.
In an acidic environment, these salts produce oxalic acid, phosphoric acid, hydrofluoric acid, boric acid, and tartaric acid. Barium oxalate, for example, reacts with HCl to produce oxalic acid.
BaC2O4 + 2HCl ⟶ BaCl2 + H2C2O4
Because these interfering acids are weak, they do not completely dissociate and remain in solution in their unionised form. Between dissociated and non-dissociated acids, equilibrium develops.
H2C2O4 ⇌ 2H+ + C2O42-
Hydrochloric acid is a strong acid and is ionised completely.
HCl ⟶ H+ + Cl-
Hydrogen ions act as a common ion among them, and a higher concentration of H+ suppresses interfering acid ionisation. As a result, the ionic product of C2O42- and Ba2+ does not exceed the solubility product of barium oxalate, and Ba2+ is retained in solution as barium oxalate. As long as the medium is acidic enough, interfering radicals do not interfere. When we add the 3rd group reagent ammonium hydroxide NH4OH to make the medium alkaline, however, OH- ions combine with H+ and neutralise them. This lowers the concentration of H+ ions, which advances the dissociation equilibrium of the interfering acid and raises the concentration of C2O42-. As a result, the ionic product of C2O42- and Ba2+ exceeds the solubility product of barium oxalate, and Ba2+ precipitates in the third group, which is actually part of the fourth.
The solution may contain one or more interfering radicals. They must be removed in the following order: oxalate and tartrate first, then borate and fluoride, silicate next, and finally phosphate.
Q18) State Qualitative Analysis of Cations.
A18) Six groups of cations are commonly used. Each group has a common reagent for separating itself from the solution. Because cationic analysis is based on the ions' solubility products, only if separation is done in a specific order can meaningful results be obtained. Because some ions from one group may react with the reagent from another group, this is the case. Both Ba2+ and Sr2+, for example, will solidify when reacting with the SO42- ion. As a result, prior to selecting the SO42- ion for selective precipitation in a solution containing both Ba2+ and Sr2+, it is necessary to perform mathematical calculations.
Example:
The concentrations of barium chloride (BaCl2) and strontium chloride (SrCl2) in a solution are 0.010 M and 0.020 M, respectively (SrCl2). Is it possible to use concentrated sodium sulphate (Na2SO4) solution to selectively precipitate either Ba2+ or Sr2+? Which ion is going to be the first to precipitate? (Assume the Na2SO4 solution is so concentrated that the volume change in the Ba-Sr solution can be ignored for the sake of simplicity.)
Solution:
The barium sulfate solubility is given by:
Ksp = [Ba2+][SO42-] = 1.5 x 10-9
With 0.010 [latex]\frac{moles}{liter} [/latex]of Ba2+, precipitation of barium sulfate will not occur until the sulfate ion concentration increases to:
[SO42-] = 0.010 = 1.5 x 10-7
Strontium sulfate will precipitate when the sulfate concentration is:
This signifies that as the sulfate ion (SO42-) is added to solution and its concentration increases, barium will precipitate first.
Q19) Explain the Effect of solubility in Common Ion Effect.
A19) In this subsection, we'll look at how the addition of a common ion affects the solubility of a salt in a solution.
The common ion effect can be used to obtain drinking water from chalk or limestone-containing aquifers (underground layers of water mixed with permeable rocks or other unconsolidated materials). The hardness of the water is reduced by adding sodium carbonate (chemical formula Na2CO3) to it.
The common ion effect is used in water treatment to precipitate calcium carbonate (which is sparingly soluble) from the water by adding sodium carbonate (which is highly soluble).
This addition of sodium carbonate produces a finely divided calcium carbonate precipitate with a very pure composition. As a result, the CaCO3 precipitate is a valuable by-product that can be used in the production of toothpaste.
Because soaps are sodium salts of carboxylic acids with long aliphatic chains (fatty acids), the common ion effect can be seen in the soap-making process. In order to reduce the soap's solubility, sodium chloride is added to the solution. However, water with a sufficient amount of Na+ ions, such as seawater and brackish water, can inhibit the action of soaps by lowering their solubility and thus their effectiveness.
Q20) Describe pH of the Common-Ion Effect.
A20) The pH of a buffer solution changes when the conjugate ion of a buffer solution (solution containing a base and its conjugate acid, or acid and its conjugate base) is added to it due to the common ion effect.
When acetic acid and sodium acetate are both dissolved in the same solution, acetate ions are produced. Sodium acetate, on the other hand, completely dissociates, whereas acetic acid only partially ionises. Because acetic acid is a weak acid, sodium acetate is a strong electrolyte, this is the case.
According to Le Chatelier's theory, sodium acetate's new acetate ions aid in the suppression of acetic acid ionisation, shifting the equilibrium to the left. The pH of the solution is raised because the dissociation of acetic acid is reduced.
As a result, when compared to an acetic acid solution, the common ion solution containing acetic acid and sodium acetate has a higher pH and is thus less acidic.
Thus, this article discusses the common ion effect, its impact on the solubility of a salt in a solution, and its impact on the pH of a solution.